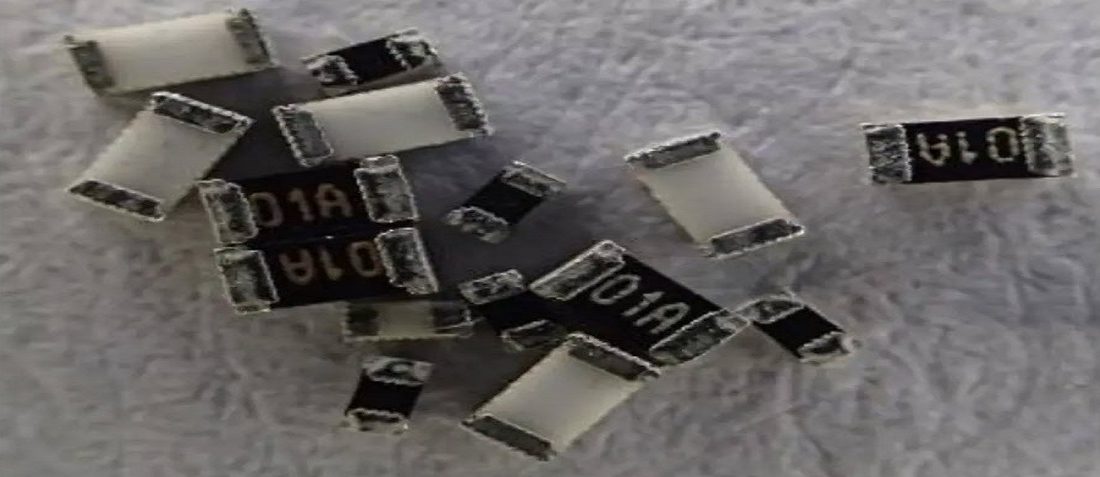
Understanding High-Precision Resistor Temperature Coefficient of Resistance
- Posted by doEEEt Media Group
- On June 23, 2023
- 0
What is the Temperature Coefficient of Resistance?
The temperature coefficient of resistance (TCR) calculates a relative resistance change per degree of temperature change. It is measured in ppm/°C (1 ppm = 0.0001%) and is defined as: TCR = (R2– R1)/ R1 (T2– T1). This specification is typically expressed in parts per million (ppm) per degrees Celsius for high-precision resistors, concerning normal room temperature, typically +25°C.
Despite the importance of this specification, individual resistor manufacturers use different methods for defining TCR on their published datasheets. In most cases, this definition does not provide enough information to enable an end user to accurately predict the influence of temperature changes on the resistance value. Of course, such published TCR variances are of concern in their potential to create measurement uncertainty, particularly in applications where high-precision resistor performance and temperature stability are absolute requirements. This uncertainty is created when there is insufficient confidence that a TCR specification has been calculated with enough data to accurately predict the true impact of temperature change on resistor performance.
For example, some manufacturers may opt to list TCR as ±5 ppm/°C or ±10 ppm/°C without reference to temperature range. Others may specify TCR as ±5 ppm/°C from +25°C to +125°C yet omit data regarding other temperature ranges. In high-precision devices like the Bulk Metal® Foil resistors manufactured by Vishay Foil Resistors, published TCR specifications include typical nominal curves, generally from –55°C to +125°C. Those curves define nominal “cold” (–55°C to +25°C) and “hot” (+25°C to +125°C) chord slopes. Their datasheets typically specify the maximum spread for each pitch (e.g., ±0.2 ppm/°C and ±1.8 ppm/°C). For a Bulk Metal Foil resistor, a default TCR interpretation of ±5 ppm/°C, for example, would mean that the resistance would not change more than +5 ppm/°C at any point across the operating temperature range.
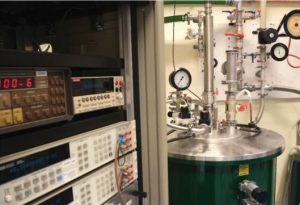
The Vishay Foil Resistors brand of Vishay Precision Group, Inc. (VPG) is longtime global industry expert in designing, developing, and manufacturing reliable high-precision Bulk Metal Foil resistors and current power sensors for diverse applications. Hundreds of standard model configurations are derived from one of the industry’s most extensive portfolios of customer-selectable housings, materials, substrates, and metal foil grade combinations. Advanced manufacturing techniques ensure that resistor designs are performance-optimized for the consistent operation to published specifications throughout their useful service life. All VPG high-precision Bulk Metal Foil resistors feature some of the industry’s most good TCR specifications, uniformly calculated per stringent industry best practices. This ensures their reliability across all resistance and operating temperature ranges.
This paper shall examine TCR and its “best practice” interpretations, as recommended by VPG’s vast experience in high-precision resistors. Overall goals shall be to understand precision resistor performance better, as it relates to temperature; to illustrate nuances among and between published TCR specifications, depending upon technology type and a manufacturer’s own chosen calculation method; and to offer further insights for TCR data utilization, as a means of ensuring that a specified precision resistor can reliably perform within its intended application.
The Relationship Between Temperature and High-Precision Resistor Performance
The impact of temperature on resistor performance is reflected internally in terms of its effects on component operation and externally in terms of resistor behaviour within the installation environment. Inherent to resistor design is the concept that, as electrical current flows through a resistor, it generates a certain amount of heat. This is a phenomenon known as the Joule effect. The thermal response created by the Joule effect then induces relative mechanical changes, or stresses, within the resistor. These stresses are caused by differential thermal expansions in the resistor materials of construction, amounts of which can vary based on the materials themselves. Ambient temperatures within the installation environment can similarly influence resistor response, generating heat, potentially affecting resistor performance.
An optimal design is, therefore, one which minimizes high-precision resistor susceptibility to external and internal stresses during different usages and power loads without sacrificing performance and reliability. In Bulk Metal Foil resistive technology, this goal is achieved by creating a precise thermo-mechanical balance between generated heat, materials of construction, and associated manufacturing processes. Through careful design, the need to compensate for the effects of heat and stress during operation can be virtually eliminated, further increasing performance stability. In recognition of the critical relationship between temperature and high-precision resistor performance, the R&D team at Vishay Foil Resistors ensures that its complete ultra-high precision resistor portfolio is designed in this manner.
For example, during the development of a Bulk Metal Foil element, a proprietary cold-rolled foil material is bonded onto a ceramic substance. That material is photo-etched into a resistive pattern without introducing mechanical stresses onto the fabric. Following this process, high-precision resistors are laser-adjusted to a specified resistance value and tolerance. Because the resistive material is neither drawn, wound, nor mechanically stressed during manufacturing, the high-precision Bulk Metal Foil resistor can maintain its complete intended design characteristics and, therefore,, total performance reliability, including TCR.
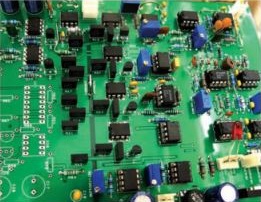
In contrast, other standard resistors manufacturing methods, such as wire winding, thin-film sputtering, or thick-film glazing, have an inherently greater likelihood of introducing mechanical stresses and, therefore, more significant potential for thermo-mechanical imbalance. End users are therefore recommended to pay close attention to rated temperature specifications to ensure that a resistor is operating according to published specifications. By adhering closely to these values, the end-user can be assured of continued resistor reliability, regardless of the manufacturing process. When a resistor operates above rated temperatures, it can fail to function or otherwise incur damage which directly compromises accuracy. If such resistor over-temperature conditions persist over an extended timeframe, individual resistance values may change permanently, leading to complete circuit malfunction. While manufacturers routinely design products with a certain margin of acceptable temperature limits beyond published specifications, such leeway can vary significantly by manufacturer.
Interpreting TCR, Chord Slopes and Change Rate Analysis Specifications
Despite differences in designs and associated manufacturing processes, TCR remains one of the most commonly accepted resistor performance stability indicators. TCR is imperative for predicting resistor sensitivity to ambient temperature variations and anticipated component behaviour at low- and high operating temperatures. As a result, the TCR of Bulk Metal Foil resistors considers extreme theoretical conditions within individual specification limits. In contrast, with other technologies, such as thin film, manufacturers typically choose to present TCR in a relatively narrow temperature range, with less emphasis or consideration of extreme temperature effects.
In addition to its previously noted definition, TCR may be further defined as a resistance change between two temperatures, divided by the temperature difference (chord slope), or TCR = (ΔR/R)/ΔT. It is common practice to define cold chord slope from –55°C to +25°C and hot chord slope from +25°C to +125°C (in this case ΔThot = 125 – 25 = +100°C). However, any other temperature interval (ΔT) may also be defined. To determine the rate of change in resistance at any temperature on that curve, TCR is calculated mathematically when ΔT becomes infinitely small (ΔT→0):
TCR(ΔT→0) = (dR/R)/ dT
It is well-known that the change in resistance vs temperature in NiCr resistors is not linear and instead normally follows a parabolic pattern. Mathematically, this function can be described by:
Y = aX2 + bX + c where: Y = ΔR/R (normally expressed in ppm)
X = T (Temperature in °C).
In this case, for any temperature T, Y will express the value of the change in resistance ΔR/R from the nominal value (at +25°C) in ppm. In other words, the derivative process Y′ will express this for the function Y. This function defines a line tangent’s slope (TCR) to the parabola and indicates how TCR changes. For the above parabola function:
Y′= 2aX + b (Y′ is expressed in ppm/°C)
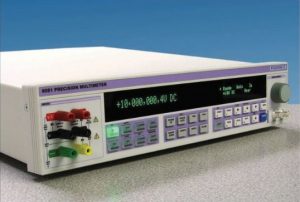
One can also use that a chord slope equals the tangent midpoint value of the relevant temperature range for simplicity. For example, the value of the hot slope (+25°C to +125°C) equals the tangent value (Y′) at the midpoint, T = +75°C.
It is a common practice for thin-film resistor manufacturers to target the best hot slope while keeping the cold pitch within the specified limit. A study comparing and analysing Bulk Metal Foil and thin-film precision resistor TCR, using the change rate calculation method, has shown that the change in resistance due to temperature can be significantly larger than specified TCR limits. This comparison is based on testing two groups of different precision thin-film NiCr resistors from other manufacturers, each identifying a TCR of 5 ppm/°C. Results of this study demonstrated that the maximum change in resistance (TCR) due to temperature changes across the temperature axis from -55°C to +125°C would vary in Bulk Metal Foil resistors from -2.17 ppm/°C to +2.2 ppm/°C for a total of less than 4.37 ppm/°C. For the same temperature range, the TCR of the thin-film resistor sample from manufacturer A will vary from -3.6 ppm/°C to +7.2 ppm/°C, for a total of nearly 11 ppm/°C; and from manufacturer B, from -9.1 ppm/°C to +4.99 ppm/°C, for a total of 14 ppm/°C. In other words, precision thin-film resistors may exhibit a TCR that is much higher than the specified limits of a manufacturer’s datasheet.
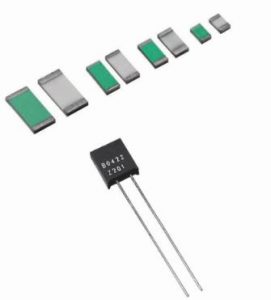
It is important to emphasize that the TCR of a Bulk Metal Foil resistor is achieved by matching two opposing effects of the inherent increase in resistance due to a temperature increase vs the compression-related decrease in resistance due to that same temperature increase. These two effects co-occur, resulting in an unusually low, predictable, repeatable, and controllable TCR specification. As a result, Bulk Metal Foil resistors achieve intrinsic maximum stability and near‐zero TCR. This specification is not reliant on screening or other artificial means for achieving uniform high-precision resistor performance and temperature stability. This stringent TCR calculation method, from the VPG experience, is employed to ensure high-precision resistor reliability across total resistance values and operating temperature ranges.
The Benefits of Low TCR in High-Precision Resistor Applications
Illustrations of the benefits of low TCR may be found within thousands of successful applications. This paper’ll review three application examples where low TCR offers certain performance advantages.
Precision Instrumentation
Transmille, a leading UK manufacturer of high-accuracy digital multimeters, sought a resistor component for a new series of 8.5- and 7.5-digit units. To achieve the necessary 8.5-digit accuracy, the specified resistor must offer extremely low TCR, high precision, repeatability, low thermal EMF, low noise, long-term stability and minimal harmonic distortion. The multimeter was based on an analogue circuit design, so the resistor needed minimal drift from initial values when operating above room temperatures. The customer selected a VPG Bulk Metal Foil resistor due to its low TCR specification of <1 ppm/°C maximum at +20°C. In addition to extremely low TCR, the resistor offered a low PCR of 5 ppm at rated power; load-life stability of ±0.005% at +70 °C for 2000 hours, or ±0.015% for 10,000 hours; a thermal EMF of <0.05 μV/°C; and non-measurable noise.
The extremely low TCR of the Bulk Metal Foil resistor allowed Transmille to introduce a new digital multimeter to the market, which could offer both industry best-in-class performance and necessary 8.5-digit accuracy. The resistor offered exceptional stability under maximum allowable drift, over thousands of hours of field service, even under harsh conditions. The user was further able to achieve this resistor performance rather cost-effectively. This allowed Transmille to introduce a new high-performance digital multimeter at a highly competitive price point to the market.
Metrology Secondary Reference
In another example, VSL, the National Metrology Institute (NMI) of the Netherlands, approached VPG for a high-precision resistor solution as a secondary reference standard in its quantum Hall resistance (QHR) experiments. QHR is the globally recognized primary quantum resistance standard, with values of around 12.9 kΩ and 6.45 kΩ. To serve as an adequate secondary reference standard, VSL needed a cost-effective, high-precision resistor, the importance of which needed to match those of the primary QHR standard closely yet offer a well-defined four-terminal configuration, low noise, low TCR and no RH effect, along with excellent long-term stability.
Based on the low TCR offered by VPG, VSL selected one of the company’s ultra-high precision resistors. The chosen resistor integrated 11 elements into a single housing, allowing for lower TCR and longer-term drift than could be achieved via a single resistive element. The device offered the necessary terminal connections, hermetic sealing for humidity protection, and oil fill, further ensuring that resistance values would remain largely unaffected by sudden temperature changes. The units were then tested for TCR value confirmations vis-à-vis the published VPG specification. For this purpose, the resistor was subsequently mounted within an enclosure thermostated at 29.00 ± 0.02°C for further reduction of TCR effects, then measured against the primary QHR over a more than five-year period. Results of the five-year study showed that the actual long-term TCR of the two Bulk Metal Foil resistive elements were less than 0.5 ppm/°C over a temperature range of +18 °C to +28 °C, with a (very) small second-order temperature coefficient Beta. This was well below the initially published two ppm/°C specification (over -55 °C to +125 °C) and proved the VPG resistors to be a reliable secondary QHR reference standard. Here, published TCR served as an added benefit in terms of its empirically proven capability to exceed published specifications under long-term use.
Diode Laser Current Drivers
In another example, low-cost and easy-to-use diode lasers are a virtual measurement staple in experimental atomic physics; careful regulation of parameters is required for a diode laser to maintain its frequency, output power, current and temperature. To best manage costs, the Physics Department at California State University looked to construct its own low-cost, low-noise current source for use with its in-laboratory diode lasers. To generate a suitably stable laser stream, the current sense resistor is needed to resist both internal and external temperature drifts, have a high-power rating and have a low thermal EMF. The most critical element of the current driver is the sub-circuit, which is responsible for current regulation since laser stability must not exceed overall sense resistor stability. In this application, using traditional commercial current controllers was too cost-prohibitive. The viable resistor solution, therefore, needed to be both low-cost and high-precision.
By using a high-precision Bulk Metal Foil resistor with a low TCR of 2 ppm/°C typical (–55°C to +125°C, +25°C ref.), tolerance of 0.01% and a 10 W power rating, the end user could integrate a regulated voltage within its sub-circuit, and yet still control the amount of current emitted from the current laser driver. The latter was achieved by tuning the driver to a set voltage on an adjustable voltage regulator. This preset total resistance value ensured that the voltage drop was significant enough for accurate current regulation, though small enough not to influence regulated supply voltage. The specific combination of long-term stability and low TCR made the Bulk Metal Foil resistor an optimal solution within a low-cost, high-precision application. The solution proved viable, as the user was assured of TCR specification accuracy.
The “True” Value of TCR in High-Precision Resistor Selection
For engineers selecting high-precision resistors, TCR specifications can help them to better predict reversible shifts in component resistance from an ohmic value within the application, both under intended operating temperatures and within the installation environment. Such data offer insights into critical longer-term resistor performance indicators and finished product designs. As TCR calculation methods can vary by manufacturer, manufacturing process, materials of construction, and other aspects, an end user needs to understand any nuances in the chosen method. This understanding, in turn, helps them better understand the value of such data as a valid component reliability metric. VPG Foil Resistor methods for TCR calculation follow strict protocols for high-precision resistors to help customers be confident in such components’ long-term reliability within demanding applications.
Source: VPG Vishay Foil Resistors
- ALTER SPACE TEST CENTER: testing approaches for New Space - September 30, 2024
- Failure Mechanism of Metallized Film Capacitors under DC Field Superimposed AC Harmonic - September 5, 2024
- Film Capacitor Technologies and Applications 2024 - September 5, 2024
0 comments on Understanding High-Precision Resistor Temperature Coefficient of Resistance