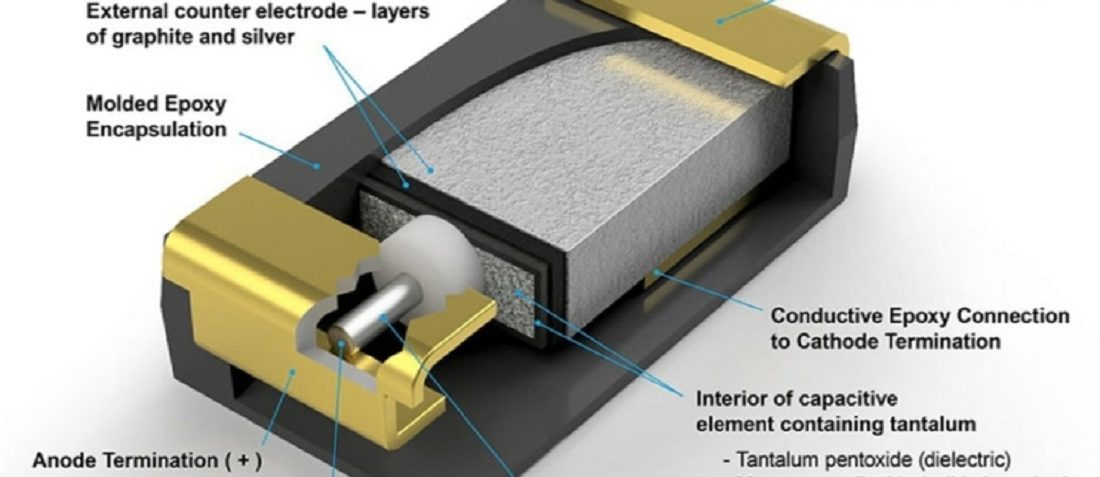
Tantalum and Niobium Capacitors
- Posted by doEEEt Media Group
- On May 15, 2023
- 0
Tantalum and Niobium capacitors belong to electrolytic capacitor types, and they are known for their high capacitance in small dimensions (high energy and power density), reliability and stability of its parameters.
Traditionally, tantalums’ advantages over aluminum electrolytic capacitors have been found in terms of capacitance per volume, parameter stability over temperature, and longevity; tantalums, in general, do not suffer from dry-out problems or issues of dielectric degradation when stored and discharged for long periods. However, tantalums are generally more costly and have a more limited range of available capacitance and voltage values.
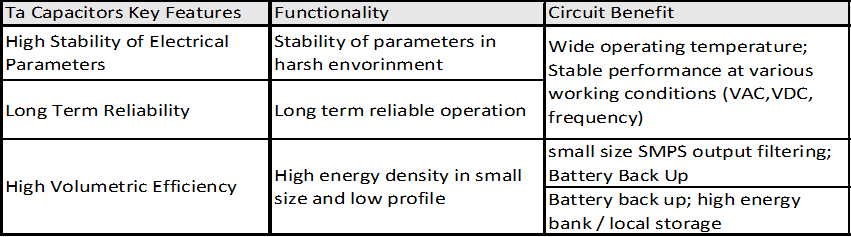
Table 1. Tantalum capacitor’s key features and benefits
The main types include:
- Tantalum solid electrolytic capacitors
- with MnO2 electrolyte
- with conductive polymer electrolyte
- Niobium and NbO solid electrolytic capacitors with MnO2 electrolyte
- Tantalum wet electrolytic capacitors
We will learn more about these types in the following chapters.
Construction and Manufacturing Process
Tantalum capacitors are manufactured from a powder of relatively pure elemental tantalum metal. Niobium capacitors from niobium metal powder and NbO capacitors from NbO powder with metal-like properties.
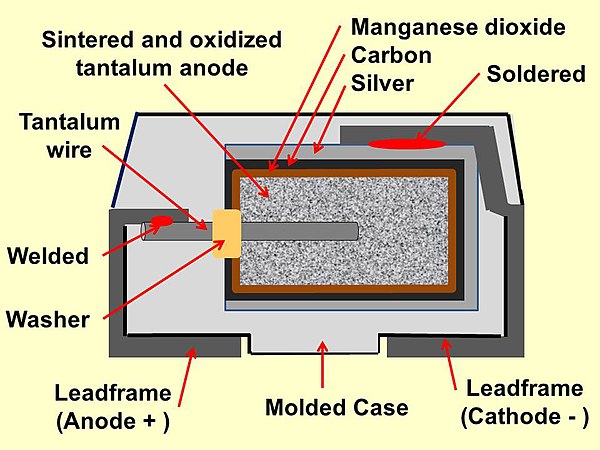
Figure 1. Construction of a typical MnO2 solid electrolyte tantalum capacitor
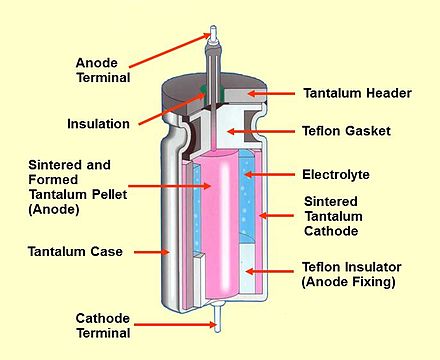
Figure 2. construction of conventional wet electrolyte tantalum capacitors
The following manufacturing process chart describes the standard tantalum chip MnO2 solid electrolyte manufacturing flow. The process is identical also for niobium and NbO chip capacitors.
The powder is compressed around a tantalum (riser) wire to form a “pellet”. The riser wire ultimately becomes the anode connection to the capacitor. This pellet/wire combination is subsequently vacuum sintered at high temperatures (typically 1200 to 1800 °C), producing a mechanically robust pellet and driving off many impurities within the powder. The powder forms a sponge-like structure during sintering, with all the particles interconnected into a monolithic spatial lattice.
The electrochemical process of anodization forms the dielectric over all the tantalum particle surfaces. The “pellet” is submerged into a fragile acid solution, and DC voltage is applied to achieve this. The final voltage applied during the forming process determines the total dielectric thickness. Initially, the power supply is kept in a constant current mode until the correct voltage (i.e. dielectric thickness) has been reached.
The next stage for solid tantalum capacitors is the application of the cathode plate (wet tantalum capacitors use a liquid electrolyte as a cathode in conjunction with their casing). This is achieved by pyrolysis of manganese nitrate into manganese dioxide in MnO2 parts (as shown on the chart) or polymerization in the case of conductive polymer types.
The top of the anode is then covered by graphite and silver paste layers for good conductivity and assembled into a lead frame. The leadframe function as a holding media for the rest of the manufacturing process – moulding, testing and ageing. In the final approach, the capacitors are cut, trimmed & formed and create their final terminations.
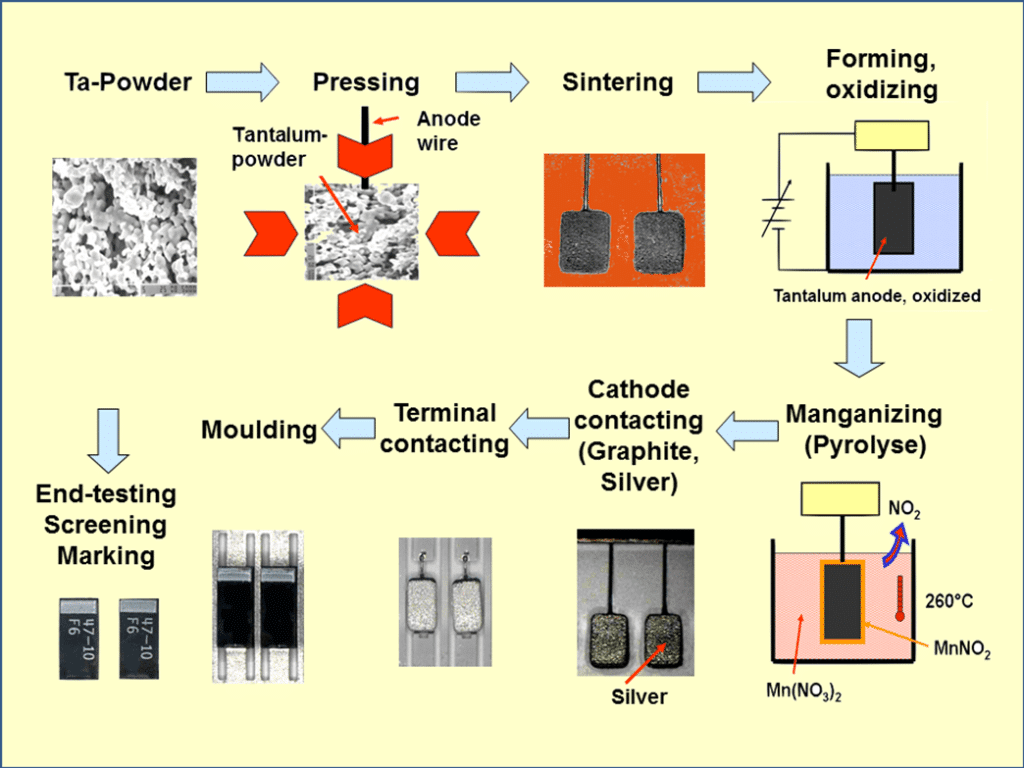
Figure 3. Manufacturing process of solid tantalum (MnO2) capacitors; source: Wikipedia
Polymer and Manganese Dioxide MnO2 Solid Tantalum Capacitors
Introduction
Cross-section schematics of typical SMD tantalum chip construction is shown in Figure 4. and 5.
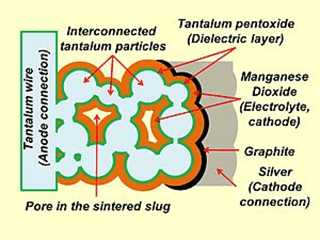
Figure 4. Explanation of tantalum MnO2 capacitor inner structure (not to scale); source: KEMET
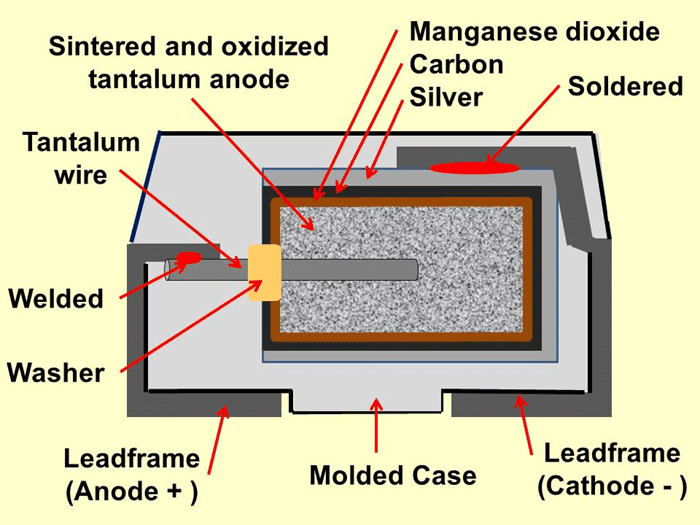
Figure 5. SMD tantalum MnO2 capacitors structure; source: KEMET
Tantalum metal has a dielectric constant of approximately 27. The price for solid tantalums is relatively high due to mainly the high cost of the tantalum powder. The solid electrolyte theoretically has no limitations in operation and storage time. The capacitance range extends up to some thousand µF. The capacitors are characterized by a high CV product per volume unit, especially when high CV powders above 200k CV/g are introduced for low and above 80k CV/g for higher voltages.
The conventional solid electrolyte is MnO2. However, conductive polymer types are more and more favourite due to their lower ESR and reduced ignition features. On the other hand, the MnO2 types are more robust against thermo-mechanical stress and stable electrical parameters under high temp & highly humid environments. Thus high reliability and more extended operation lifetime applications still use mainly established reliability MnO2 types as the main tantalum solid capacitor technology.
Physically, the capacitor also has a high density. Thus, lead-mounted components permanently should be fixed mechanically against their substrate. This type requires large margins to the forming voltage, usually 2 to 4 times VR. Ratios higher than four are needed for automotive and other high-reliability applications, while for consumer electronics, a balance around two may be sufficient. The higher ratio favours not only higher reliability but also better capacitance stability.
On the other hand, higher VF/VR ratios mean a correspondingly lower volumetric efficiency (capacitance is inversely proportional to the dielectric thickness). The maximum VR stops at 125 V DC for capacitors with polymer electrolytes and 100V DC for MnO2 types. However, derating rules are different. It is recommended to derate MnO2 types by 50% for current surge circuits (directly on battery or DC/DC input) and 20% for non-high surge existing applications (DC/DC output, timing, coupling/decoupling …). Tantalum polymer derating is recommended at 10% for up to 16V capacitors and 20% for >16V capacitors for all kinds of applications.
The capacitance stability is good (∆C/C ≤ ± 5%), and the tolerances range from (±5) ±10% to ±20%. The reverse voltage should, at a maximum of 85 °C, be limited to the most negligible value of the alternatives: 10 % of VR or 1V. Continuous reverse voltage operation is not recommended. However, small uneven spikes, such as diode overshoot voltage, are acceptable. Chip designs are becoming very common and well documented; some basic case sizes have been established with a functioning international standardisation. However, the market need and manufacturers’ feedback was faster thann standardization. Theree areseveralf other case variants with different letter codes per manufacturing that may be very confusing in some cases – as the same letter from one manufacturer has different dimensions to the other.
For example,, AVX X case is a 1.5mm low profile variant of the standard D case 7343 chip (the AVX X case is equivalent to KEMET’s W case). However, KEMET X case is a taller 4.3mm variant of the classic D case 7343 part. (KEMET X case is equivalent to AVX E case).
The case size variants from manufacturers on the market is, so vast that they are now using nearly complete alphabet options and have begun to use also numbers or combinations of letters and numbers for different case size dimensions. The following table shows the standard few sizes of a series that has acquired international acceptance under the same letter code:
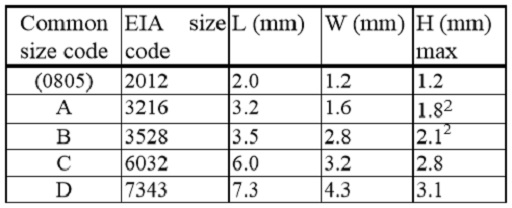
Table 2. Standardized SMD tantalum capacitors chip sizes.
SMD Chip Construction Variants
The most common construction design shown in Figure 5. is called the J-lead type as per the termination shape. There are also some other construction designs on the market – see Figure 6. below.
- J-lead – the most common, cost-effective tantalum capacitor design with J-lead robust termination that works like a spring absorbing mechanical shocks and vibrations
- Undertab (facedown) – termination placed at the bottom of the case. This allows high-density board mounting (no solder on capacitor sides) and maximises its energy density / maximum capacitance and CV by inner space for the larger anode. It does not allow solder quality visual inspection, so high-rel applications do not prefer this solution.
- Diced design – such as TACmicrochip in the figure below – proprietary AVX construction combining tantalum wafer and dice through technology allowing miniaturization down to 0201 case size. Diced designs are also available from other vendors such as Kemet/NEC or Vishay – most in combination with under tab configuration to maximize its CV.
- Conformal – original Spraque design – Vishay/Nichicon (AVX now) of dipped-coated tantalum anodes. The advantage is in large, high CV anode; the unmolded package is not so flat with potential high-speed pick and place issues.

Figure 6. SMD chip tantalum capacitors packaging types; source: AVX
Failure modes, Self-healing, Burn-in, Acceleration Factor (AF) and Derating
At first it has to be said that tantalum capacitors have been developed are representing one of the most reliable capacitor technology, historically linked with high rel applications. Nevertheless, solid tantalums sometimes are randomly stricken by short circuits. If the circuit has a high impedance it may happen that the failure disappears. A kind of self-healing has occurred. This is called sometimes a “scintillation”. Under certain conditions, this kind of self-healing may occur also in circuits where the impedance is low. The reasons for this type of failure have been the subject of comprehensive research and tests. After forming the oxide film contains a number of weak sites caused by impurities in the tantalum metal or at sites of crystalline defects. If a voltage is applied gradually, these sites will be the place for local higher currents that generated heat and heal the defect site. This is true for both MnO2 and conductive polymer while the mechanisms and effectiveness are different.
The local current generates heat that creates temperatures in the micro spot of 400 to 500 °C. At that temperature the manganese dioxide in the case of MnO2 types is reduced to a lower degree of oxide (Mn2O3) with several orders of magnitude higher resistivity. An insulating “patch” is formed over the leakage site, the local leakage current is choked and the capacitor self-heals. This type of self-healing is provoked during the production phase by a voltage load, a so called voltage aging, that for every eliminated leakage site successively forces the leakage current down.
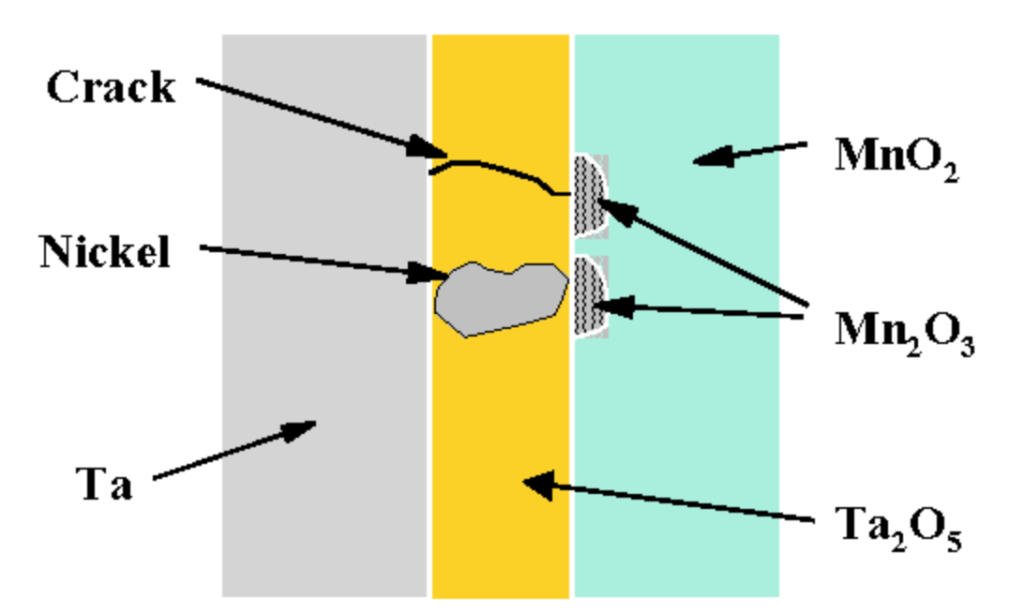
Figure 7. Tantalum MnO2 capacitors self-healing process
MnO2 self-healing considerations:
- Microscopic localized mechanism with “no” influence on Capacitance, ESR
- “no” impact on DCL
- 100% hard surge current screening mandatory for ESA/MIL
- self-healing and capacitor recovery may be limited by a combination of the following factors:
- high circuit resistance circuits >100kOhm
- aggressive reflow (= greater thermal damage to dielectric)
- very high derating>70%
MnO2 Tantalum Capacitors Design Considerations
While the tantalum self-healing process is bringing high reliability for the MnO2-based tantalum capacitors, the ignition, if overloaded, poses an essential point for carefully evaluating its circuit applications. The following are general guidelines regarding the application of Ta-MnO2 capacitors:
- Use series resistance – if applicable: limiting the external current to a fault dramatically reduces the chance of a fault site reaching the critical ignition temperature. Historically a series resistance of 1 to 3 ohms per applied volt has been recommended; this is no longer required. Modern designs may not tolerate this much ESR, and larger devices may contain sufficient electrical energy when charged to self-ignite should a fault suddenly appear. In these cases, derating and device screening are essential.
- Derate voltage: Derating is the most effective way to increase steady-state reliability; derate to 50% of rated voltage and as much as 70% when series resistance is meager, on the order of 0.01 ohm per applied volt or less. If currents are externally limited – in typical/no surge applications, 20% derating may be sufficient.
- Burn-in carefully: Many tantalum failures occur on the first power-up of an assembled device due to assembly-induced dielectric faults. Facilitating successful self-healing through the gradual voltage application via a current-limited source may avert some of these failures. Subsequent exposure to maximum expected electrical and environmental stresses will serve as a proof test since Ta-MnO2 capacitors that survive a given set of pressures once are likely to survive them almost indefinitely.
- Limit transient current: Current flows over the manufacturer’s stated surge current limit are to be avoided, including those arising from non-routine events, such as hot plugging of batteries or power supplies, short-circuit faults of system outputs, etc. Without a current surge specification, a value Imax<Vrated/(1+ESR) has been suggested.
- Observe ripple current/temperature limits: Ripple current ratings are typically based on the amount of ripple required to produce a given rise in device temperature above ambient. Excepting cases where the resulting waveforms would violate voltage or surge current limits, ripple current limits are a thermal management issue. Evaluate the test conditions under which the datasheet ripple limit figures are specified, and adapt those limits according to actual application conditions.
Polymer Capacitors Self-healing and Derating
There is some self-healing effect also on tantalum polymer types as due to the high spot temperature the conductive polymer may evaporate/peel off (delaminate) or oxidize to high insulating state, thus the failure spot is eliminated. In effect, the capacitor reduces its high leakage through the defective site. In comparison, the self-healing on MnO2 is still considered as more effective, especially at lower currents. On the other hand, the self-healing in MnO2 parts is releasing some free oxygen that may extend the ignition failure mode. This is also one of the reasons for higher derating (50%) of MnO2 types for high current circuit applications.
Polymer capacitors are much more robust against ignition and surge failures compared to MnO2 types. It is thus also reflected in its application derating recommendations usually as low as 10% up to 16V capacitors and 20% for >16V. See below the applied voltage vs component-rated voltage recommendation table:
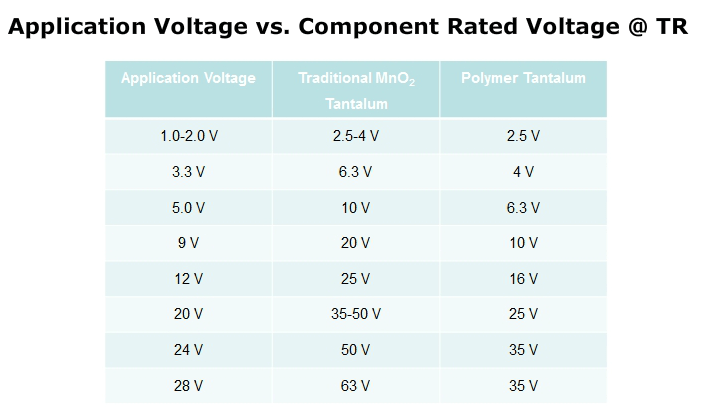
Table 3. Tantalum capacitors derating table
polymer self-healing by evaporation
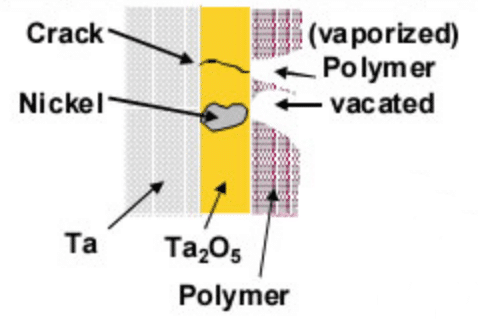
Figure 8. tantalum polymer capacitor self-healing mechanisms
polymer self-healing by oxidization
ESR reduction
ESR can be reduced by changing of MnO2 solid electrolyte to a more conductive polymer material, however, there are also other ways to reduce ESR by change of the construction and anode shape.
One way to reduce the ESR is to shorten the current path to the capacitor elements in the centre of a pellet. The following schematic of different designs shows several methods to achieve this. In Figure 9. design example No. 2, the rough surface of the coated anode is covered with the outer silver paint. Compared to the conventional anode in design No. 1 the average distance between the equipotential silver paint plain and the dielectric is reduced which means a reduced ESR.
A further ESR reduction is achieved by dividing the tantalum pellet in several elements that are connected in parallel – so-called multi-anode technology – where the current paths are reduced considerably and the conductive paint surface is even larger than in design No. 2. The method is introduced in recent years and will certainly be developed further. Roughly the ESR is inversely proportional to the number of elements in parallel.
Note: When improvement of “ESR” is mentioned, we mean ESR value measured at standard measurement conditions = 100kHz “high frequency” important for SMPS output capacitor performance. The multiple anode or rough anode design is in fact using a “skin” effect that improves ESR at higher frequencies while ESR at lower freq. such as 120Hz is not impacted or slightly higher as it is driven mostly by dielectric losses.
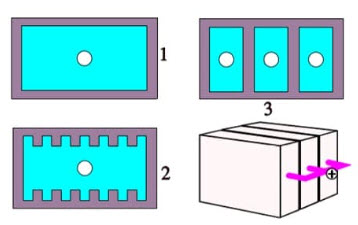
Figure 9. Schematic of ESR reduction by different design improvements. 1. Conventional anode design. 2. Macroscopic rough anode surface (flute design). 3. Multiple anode design by inner parallel connection of individual capacitor elements.
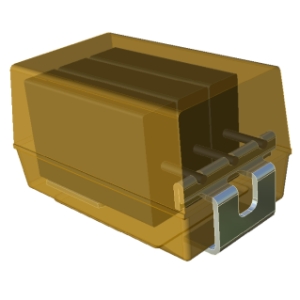
Figure 10. SMD tantalum multianode construction; source: AVX
The ESR (skin effect) depends also on the layers coating the manganese dioxide electrolyte or a conductive polymer. First the buffer between the electrolyte and the silver paint, i.e., the carbon layer, then the silver paint that is bonded to the leadframe by a silver adhesive. Layer thickness and distribution, material and treatment during production all contribute to a low ESR that has to remain low even after environmental exposure.
Reverse Voltage
Solid tantalum electrolytic is considered as being able to stand more reverse voltage than wet electrolytes. The diode function is in other words, not equally evident. The diode in the equivalent circuit ought to be supplemented with a Zener diode. What, then, the Zener voltage is varies according to certain short-time investigations from 15 to 40% of VR, depending on lot and manufacturer. Long-term investigations point to more conservative values. This is underlined by the fact that advanced powder technology has increased the sensitivity to reverse voltages. Some leading manufacturers may have different recommendations for reverse voltage. If nothing is said about reverse voltage limitations, we should, for high-reliability applications, choose the least of applicable values from the following alternatives:
Solid Tantalum Capacitors Properties
Capacitance versus temperature

Figure 11. Typical curves for capacitance versus temperature in solid tantalum electrolytic capacitors for MnO2 and polymer types.
Capacitance versus frequency
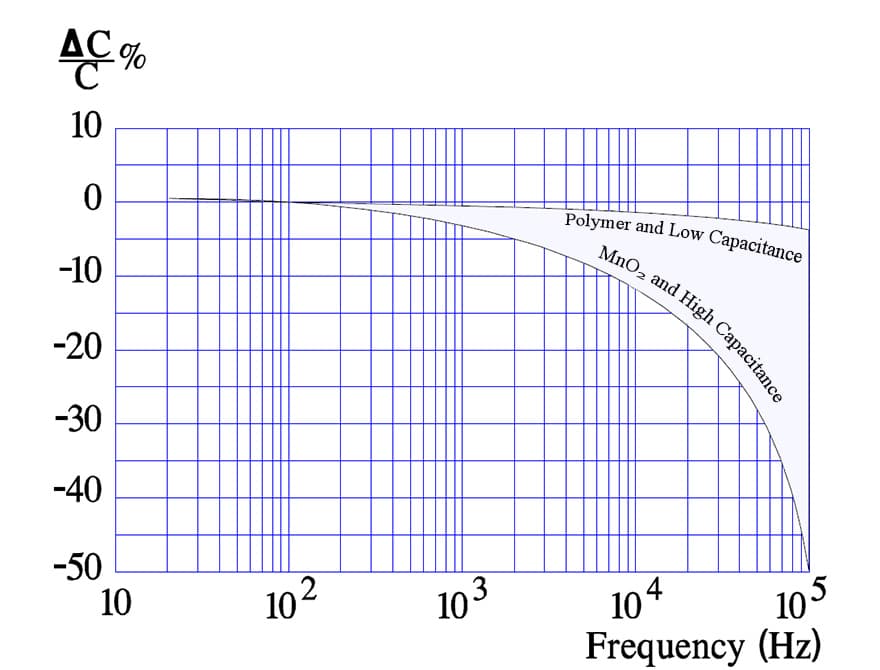
Figure 12. Typical curves for capacitance versus frequency in solid MnO2 and polymer tantalum capacitors
The higher the porosity and the finer the powder, and the worse conductivity (MnO2), the sooner the capacitor starts losing capacitance due to the increasing time constant of the farthest localized capacitor elements in the pellet. On the contrary, when the ESR is reduced, the time constants decrease. That means the so-called capacitance roll-off is moved toward higher frequencies, and the capacitance decrease will be correspondingly smaller. The effect of multianode is demonstrated in the MnO2 part, which shows examples from 470 µF / 6.3 V tantalums of different designs. The impact of multianode construction for polymer tantalum is not such a big change in capacitance vs frequency characteristics (as it is already more-less flat), but to the absolute ESR value.
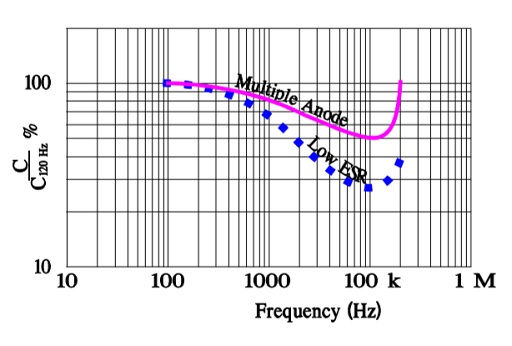
Figure 13. Comparison diagrams of the frequency influence on capacitance in multiple anodes and low ESR designs on MnO2 tantalum capacitors
There is practically no ESR temperature dependence on polymer electrolytes and small dependence on manganese dioxide electrolytes – see Figure 14.
ESR versus temperature
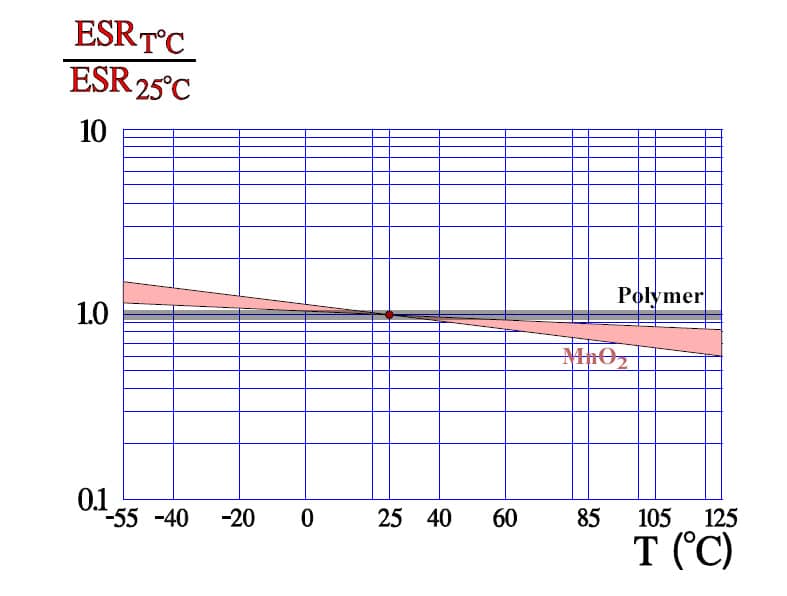
Figure 14. Normalized ESR versus temperature for MnO2 and polymer tantalum capacitors. Reference: ESR at 25 °C
ESR versus frequency
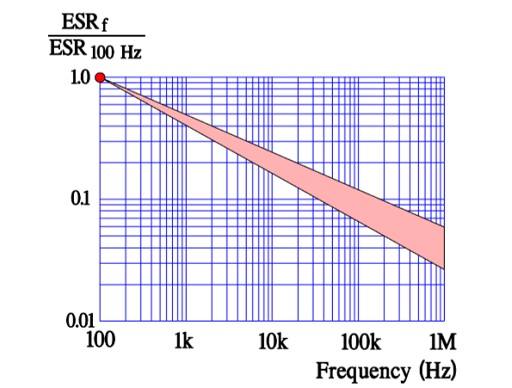
Figure 15. Normalized ESR versus frequency on MnO2 and polymer tantalum capacitors. Reference: ESR at 100 Hz
Tan δ DF versus frequency
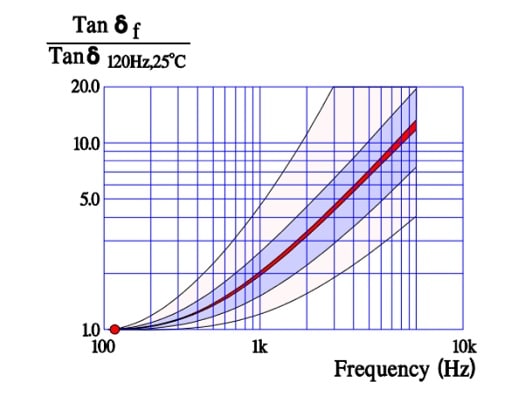
Figure 16. Normalized Tan δ versus frequency at 25 °C for MnO2 and polymer tantalum capacitors. Reference: Tan δ at 120 Hz
Note the difference in the frequency dependence of the two-loss characteristics ESR and Tan δ in Figures 15. and 16. The influence of the linearly decreasing ESR in Figure 15. is visible at lower frequencies in Figure 16. but becomes more and more negligible when the frequency increases, just as the formula predicts: Tan δ = ESR x ωC.
DCL Leakage current versus ambient temperature
The MnO2 DCL specification limit for standard parts is usually given as follows:
DCL = 0.01 x CV
,where C=capacitance, V=rated voltage … thus 100μF 10V MnO2 capacitor has a DCL standard limit as 10μA at rated voltage. In practice, by the rule of thumb, the typical leakage current is about ten times lower, so in real measurement, we can expect DCL to be around 1μA for the 100μF 10V part.
The basic DCL leakage current level on MnO2 capacitors is typically 10x lower compare to the polymer capacitors. Thus for polymer tantalum capacitors, DCL specification limits follow:
DCL = 0.1 x CV
The 100μF 10V polymer capacitor has a DCL standard limit as 100μA, and typically, we can measure around 10μA at room temperature.
An important note is that due to the continuous improvement, leakage current limits may be specific to the series and manufacturer. Low leakage series or consumer highest CV series (with higher DCL) are available on the market that differs with DCL specification limits to the above general equations. It is always a good idea to check the manufacturer’s specification datasheet.
Normalized DCL leakage current plot as shown in Fig 17. is valid for both MnO2 and polymer types. However, the absolute DCL values differ!
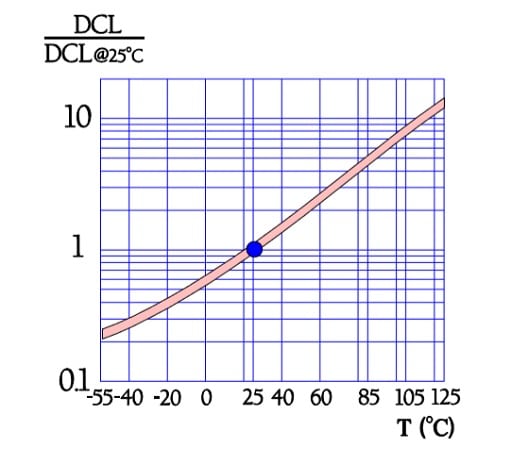
Figure 17. Normalized solid tantalum capacitors leakage current (DCL) versus temperature
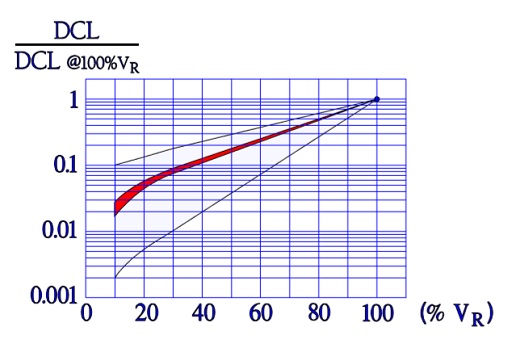
Figure 18. Normalized solid tantalum capacitors leakage current versus voltage in per cent VR
Leakage current versus voltage
The Figure 18. above shows a drop of the DCL with applied voltage (valid for both MnO2 and polymer types). By the rule of thumb, DCL drop in one range with 50% derating. So taking an example of the 100μF 10V MnO2 capacitor with a DCL limit of 10μA at 10V rated voltage, we can assume the maximum leakage current values as 1μA at 5V applied, and typical values would be around 0.1μA.
Leading manufacturers are now providing high-fidelity simulation tools where electrical characteristics of CAP, ESR, IMP, DF, DCL and S-parameters can be followed for individual capacitor part numbers.
Solid Niobium and Niobium Oxide Capacitors
Niobium is a sister metal to tantalum and shares many chemical characteristics with it, in addition to a few disadvantages and advantages of its own when used as a capacitor dielectric.
Niobium capacitor technology has existed for decades. Its limitation in maximum rated voltage, lower volumetric efficiency, incompatibility with low ESR polymer electrodes, and limited range and several vendors keep this technology as a niche line of the tantalum capacitor industry today. On the other hand, some advantages (such as lower cost, safety and reliability) are still worth a closer look and consideration.
Brief History
Capacitor manufacturers have evaluated niobium material for years every time the tantalum supply situation becomes unstable. Its lower cost base was also evaluated to replace low-voltage aluminium electrolytic.
Development of the first niobium capacitors started in the former USSR already in the 1960s, motivated by a shortage of tantalum there. However, at that time, niobium powders were unavailable in appropriate low-impurity, high-quality levels, and the capacitor reliability was inferior to the tantalum capacitors.
Niobium-based material, as it is easier to get and more abundant in nature, captured the attention of most of the leading tantalum manufacturers, especially after the tantalum shortage and supply chain issues around the year 2000. Besides niobium metal powder, J.Fife has introduced NbO material with metal conductivity level by J.Fife (U.S. Patent 6,391,275 B2), resulting in some advantages for capacitor application properties. Upon the new development and availability of low impurity Niobium/NbO powders within the years 2001-2003, companies AVX, EPCOS (tantalum division acquired by KEMET in 2007), Hitachi (bought by Holy Stone in 2009, acquired by Vishay in 2014), KEMET, NEC and Vishay announced some early sampling and preproduction of niobium-based capacitors in 2002.
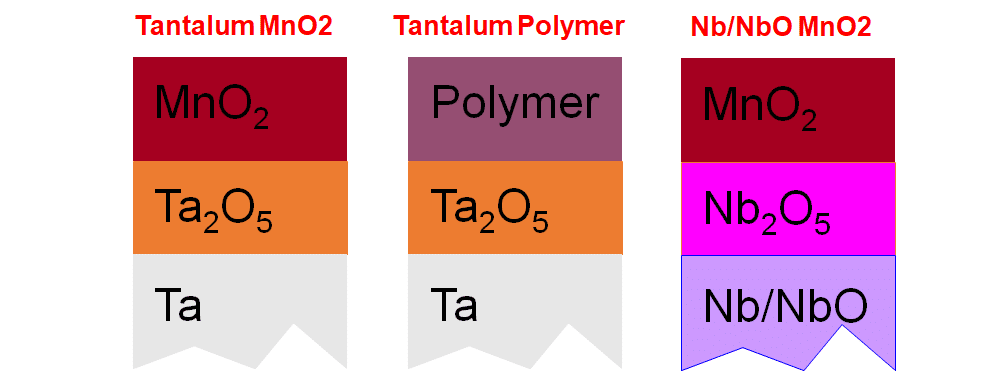
Figure 19. Tantalum MnO2 / polymer and Nb/NbO MnO2 capacitors structure
Construction of SMD chip niobium capacitors is identical to tantalum, including the manufacturing process route. Niobium or Niobium oxide powder is used instead of tantalum, and the dielectric is Nb2O5 instead of Ta2O5 see Fig. below. The dielectric constant of Nb2O5 is 41 ~ twice higher compared to tantalum pentoxide, however its density and electrical breakdown strength is about a half. This does not bring the CV advantage of Nb/NbO capacitors over tantalum; however, as the dielectric has to be ~ twice thicker than tantalum, it benefits stability and reliability.
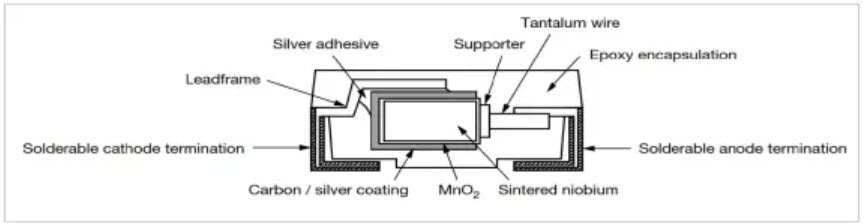
Figure 20. NMC series of niobium-based capacitor structure; source Vishay
Nevertheless, despite the very optimistic first results, niobium posed some early technical challenges that capacitor makers struggled to overcome. The obstacles included a high dc-leakage current (two to five times worse than for tantalum capacitors), an increase of DCL with lifetime and susceptibility to damage by thermal shock during the reflow manufacturing process. Low-CV capabilities have also been a problem because of the lack of high-grade, high-purity capacitor-grade powders at that time.
The Tantalum supply chain has consolidated in further years, and the urgency for niobium-based capacitor development dropped versus requirements for lower ESR tantalum capacitors with conductive polymer electrodes. In co-incidence, conductive polymer junction to Nb2O5 dielectric is resulting in even higher DCL that practically disqualifies its use with niobium-based capacitors. This next technical challenge would require additional development resources to address some basic physics issues related to the potential development of polymer niobium-based capacitors. Hence, most of the manufacturers terminated their further development effort, focusing their development power to other priorities and obsoleted their niobium-based capacitor lines.
Niobium-based capacitors with MnO2 solid electrolytes are still on the market with high reliability versus cost-unique value in certain applications. Vishay obsoleted its SMD chip niobium capacitors in 2017, and the major supplier of SMD NbO capacitors today is AVX Corporation which dedicates its product to mainly automotive, aircraft, defence and high safety applications. Axial niobium capacitors are also still made in Russia by the company OJSC ELECOND for industrial, defence and highly reliable applications.
Niobium-based Capacitor Technology and Features Background
Volumetric efficiency
Niobium, as mentioned, is in many ways behaving very similarly to tantalum capacitors. Niobium or NbO material can be processed into a form of powder with a stable oxide Nb2O5 (vs Ta2O5 in the case of tantalum) as high dielectric constant insulation. Niobium pentoxide has a dielectric constant about twice as high as that of tantalum oxide. However, the density of niobium is only half that of tantalum, resulting in similar or slightly lower volumetric efficiency compared to tantalum capacitors.

Table 4. Characteristics of the different tantalum and niobium capacitors oxide layers; source: AVX
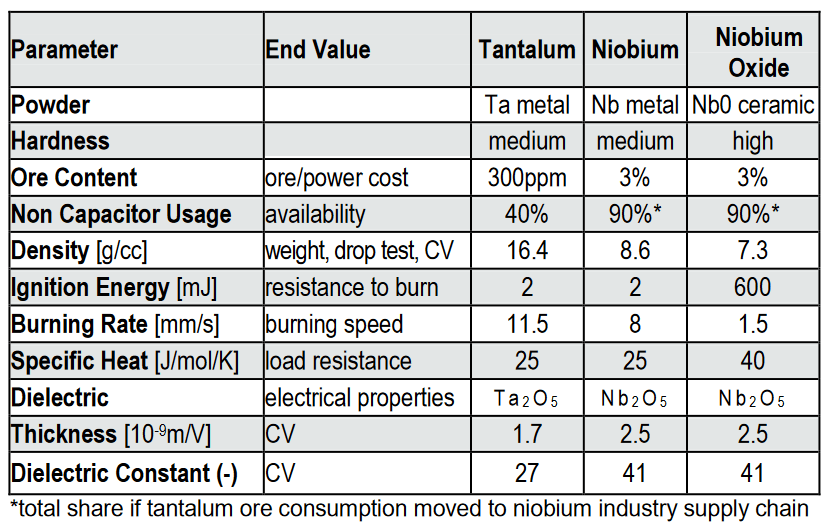
Table 5. Tantalum vs Niobium vs Niobium Oxide features; source: AVX
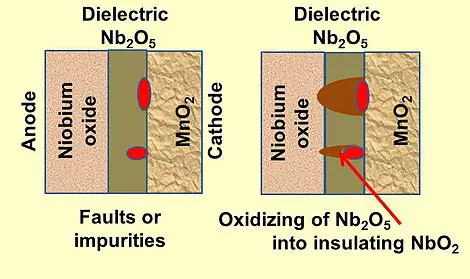
Figure 21. Self-arresting mechanism of NbO capacitors
Operating temperature:
Another difference to tantalum capacitors is that Nb2O5 dielectric is more sensitive to higher temperature operation above 85°C. Reliability up to 85°C may be considered as better, about equal at 105°C and inferior at 125°C requiring higher derating compared to tantalum capacitors.
The natural high-reliability performance of niobium-based capacitors up to 85°C (including safety margin) makes them suitable for high-performance industrial, automotive, defence, medical support, and aerospace applications, even at safety-critical applications.
Rated voltage:
As mentioned, the density of niobium is only half that of tantalum, so twice as much material is needed per unit volume to provide the same charge. The Nb2O5 dielectric constant is higher, but you must form a thicker niobium oxide dielectric for the same voltage. That is good to reduce further the electrical field stress to the dielectric (and achieve better reliability) on one side, but there is a certain limitation in maximum dielectric thickness / maximum rated voltage, driven by powder impurities and formation techniques on the other site. As a result, niobium-based capacitors feature significantly lower maximum rated voltage compared to tantalum capacitors.
Limitations
One limitation of niobium-based technologies is a lower maximum rated voltage (~10-16V) and lower CV in comparison to conventional tantalum capacitors with MnO2 (still higher compared to other capacitor technologies).
As the tantalum supply chain consolidated, niobium-based technology has been ceased by a number of manufacturers, thus limited sources and product range are the main current issues of the technology apart from the technical borders discussed above. Case sizes, mounting guidelines etc of the niobium-based chip capacitors are, however, identical to tantalum and tantalum polymer capacitors, which can be considered as drop-in alternatives on the same footprint to avoid single-source issues. Thus, the niobium-based capacitors can be considered as an additional option where tantalum capacitor designs are considered with enhanced safety features.
Properties
In comparison with solid tantalum, the temperature dependence is slightly higher.
Capacitance versus temperature
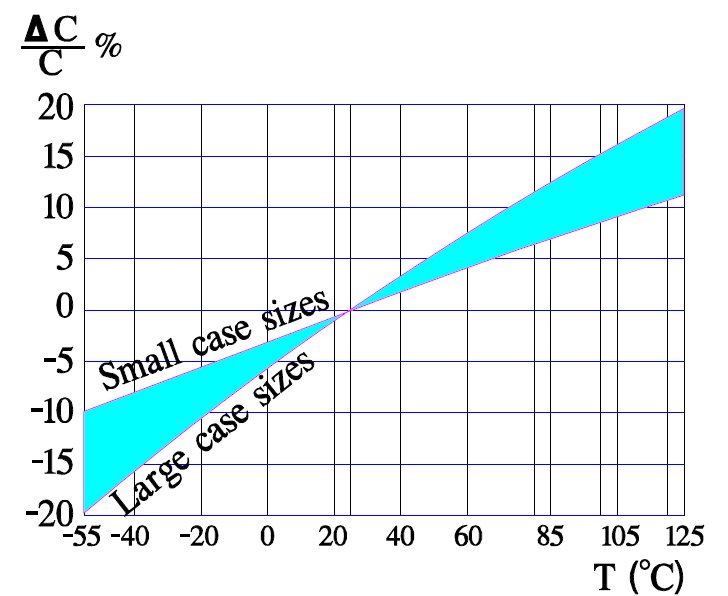
Figure 22. Typical curves for capacitance versus
temperature in solid niobium electrolytic capacitors.
Capacitance versus frequency
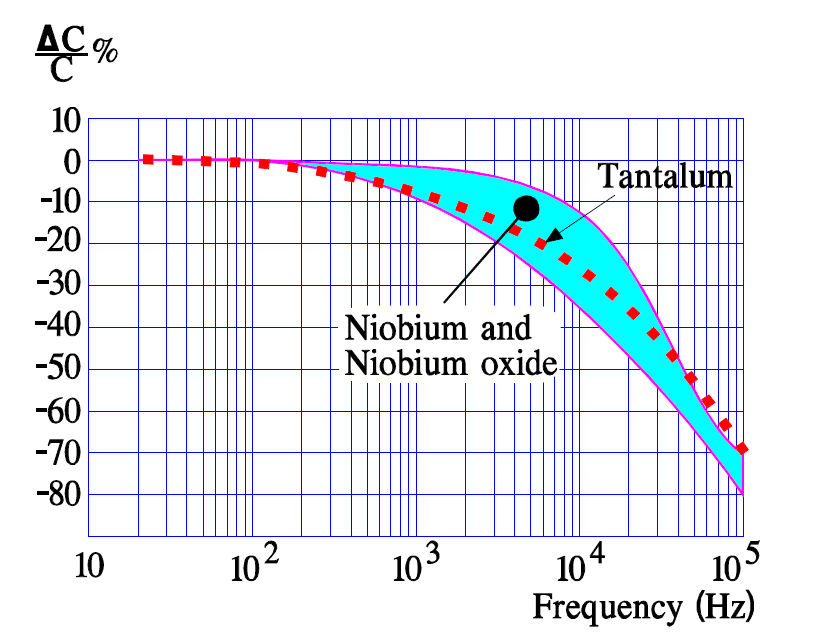
Figure 23. Diagram examples of capacitance versus
frequency in solid tantalum and solid niobium/niobium
oxide electrolytic capacitors of the same voltage and capacitance ratings
The higher the porosity and the finer the powder, the sooner the capacitor starts losing capacitance due to the increasing time constant of the farthest localized capacitor elements in the pellet. Because niobium powders have a slightly higher porosity than tantalum, their frequency dependence is correspondingly higher, as seen in Figure 23. above.
ESR versus temperature
Because the MnO2 counter electrode dominates ESR, we may expect approximately the same behavior as in solid tantalum capacitors.
The ESR in niobium / NbO electrolytic capacitors is slightly higher than that of solid tantalums at frequencies around 100 Hz. The difference decreases successively and is at 1 to 10 MHz negligible.
ESR versus frequency
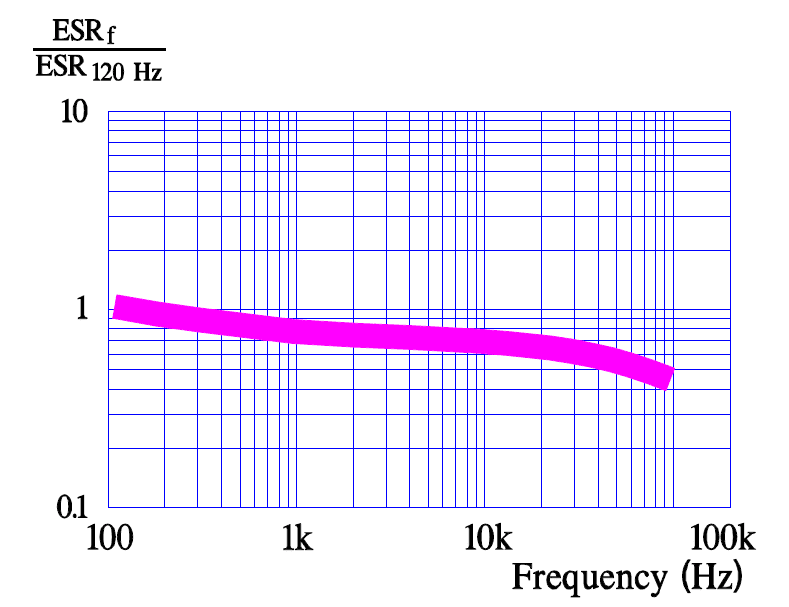
Figure 24. Typical ESR versus frequency diagrams for
tantalum and niobium/niobium oxide electrolytic capacitors based on
100 uF/6.3 V components
Leakage current versus ambient temperature
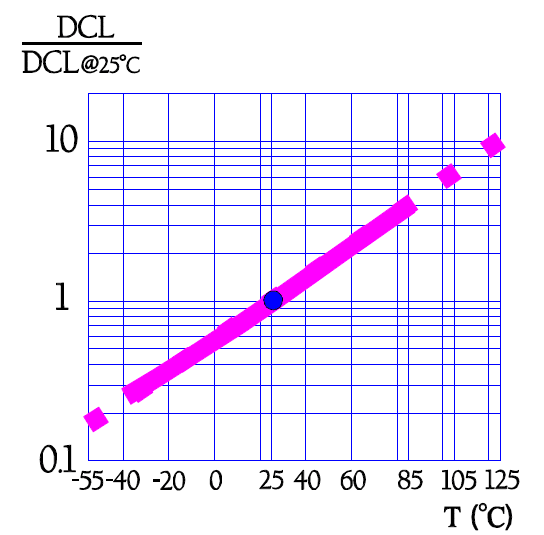
Figure 25. Normalized leakage current (DCL) of niobium electrolytic capacitors versus
temperature. Reference: DCL at 25 °C
Applications
Where the niobium capacitors bring their benefits:
- High reliability, long-term operation, safety circuits, with “standard ESR requirements” up to 8V application voltage operating in an environment up to 85°C safety (105°C qualification) with a little more space on board needed compared to tantalum capacitors (still smaller than aluminium electrolytic). The parts show high mechanical robustness against shocks and vibrations and stable electrical performance (over MLCC class II capacitors).
There is a wide range of applications where niobium-based capacitors can bring their benefits in low cost for high safety and reliability standards.
Applications with benefits from the downsizing of aluminium electrolytic capacitors:
- Consumers such as home theatres, game controllers, and white goods controllers.
high value in lower cost versus safety and reliability
- Industrial with enhanced safety features such as smoke detectors, security electronics etc
- Automotive, such as cabin electronics and telematics
- Aircraft – on board entertainment, telematics, defence (COTS+ version available)
Summary
Niobium-based capacitors present a niche capacitor market share within the tantalum capacitor industry. Nevertheless, some of its unique features, especially high safety, no short circuit failure mode under standard operating conditions and high reliability, bring its value to the end users to remain as a viable capacitor solution in the longer-term future.
The NbO capacitor, within its rated voltage, is not likely to fail as a short circuit in applications that, makes it one of the safest capacitor technologies on the market at the class of up to 8V operation voltage – counting with 20% derating of its maximum 10V range. The main applications are then 3.3 and 5V lines where safety is the prime focus, such as aircraft or automotive electronic hardware.
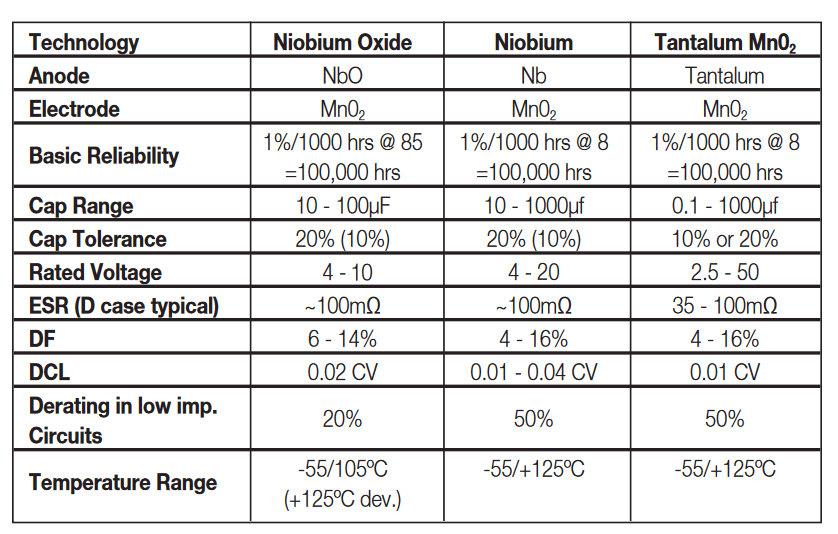
Table 6. NbO vs Nb vs Tantalum solid electrolytic capacitors parameters; source: AVX
Wet Electrolytic Tantalum Capacitors
Introduction
Wet tantalum capacitors have several advantages over solid tantalum, aluminium electrolytic, and ceramic capacitors. As with all other capacitors, these advantages lead to a very specific “sweet spot” or focused area of applications where the wet tantalum capacitor is the best and preferred choice. Wet tantalums require little derating and offer higher voltages (75 V, 100 V, and 125 V) than solid tantalums.
Rating for rating, wet tantalum capacitors tend to have as much as three times better capacitance/volume efficiency than aluminum electrolytic capacitors. With their hermetic case designs, wet tantalums have a very long life and low DC leakage. Fig. 26. below shows where the wet tantalums fall within the capacitor families. As shown, they offer higher capacitance and voltage than solid tantalums, but smaller sizes than aluminum devices with the same rating.
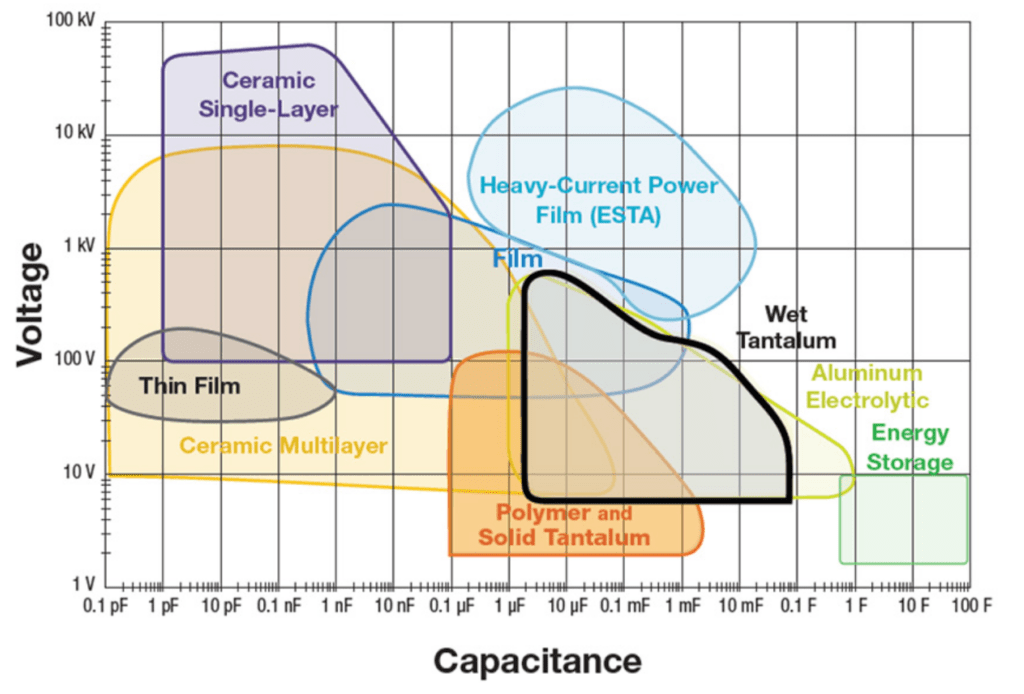
Figure 26. Wet tantalum capacitors CV position versus other capacitor technologies; source: Vishay
As we already have indicated, there are two most common designs of wet tantalum electrolytic with different cathode designs: the sintered case and the proprietary cathode design style. In history, there were one more style – foil tantalum wet capacitor that existed either with a plain or an etched anode foil. Because this design was expensive, relatively unusual and normally can be replaced with a wet aluminum electrolytic we will just mention its existence.
A typical construction of a sintered design with a tantalum case MIL-C-39006 (left) and proprietary cathode DLA 93026 (right) may look like the one in Figure 27.
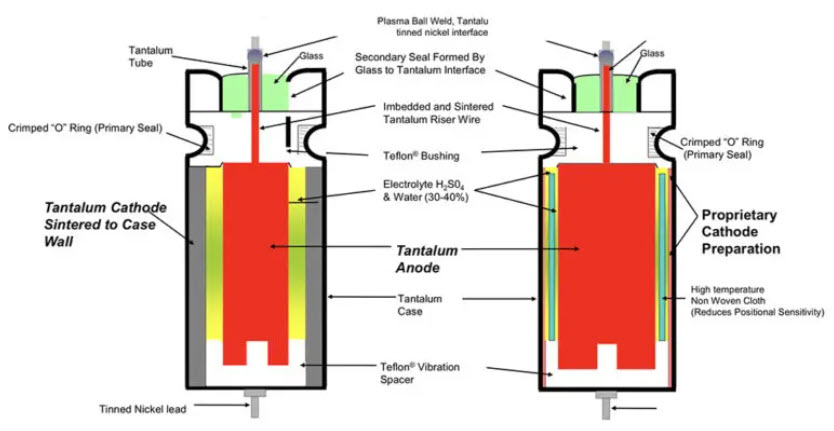
Figure 27. cross cut of wet tantalum capacitors designs conventional MIL-C-39006 design (left) and proprietary cathode high CV DLA 93026 design (right); source: Vishay
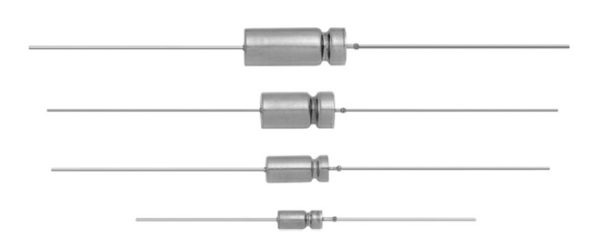
Figure 28. standardized wet tantalum cases
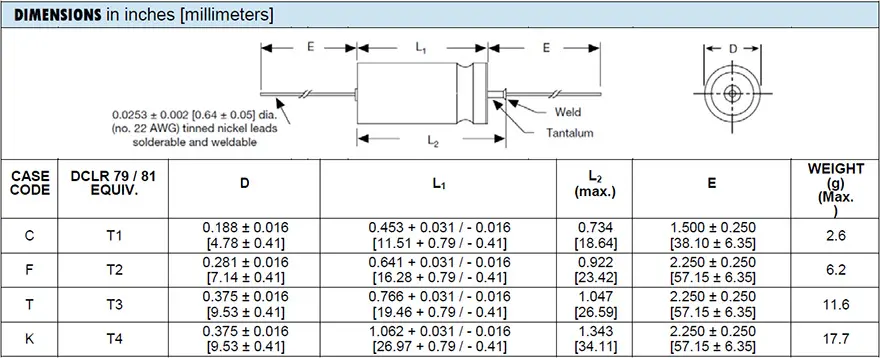
Table 6. Standardized wet tantalum cases and dimensions
The wet sintered tantalum electrolytic with a proprietary cathode system has the highest CV product per volume unit of all polarized electrolytic. On the first hand, it depends on the formation voltage that, compared to solid tantalums, is approximately three times lower. This means that the corresponding dielectric thickness of a certain rated voltage will be only one-third of that for the solid one, and the capacitance will be three times greater. Thus, the solid tantalum capacitor needs a surface three times larger than that of the wet one to get the same capacitance.
The wet-sintered tantalum capacitor also has the lowest leakage current of the electrolytes. It is comparable with that of paper capacitors.
Exactly as with solid tantalums the density is so high that lead-mounted types should be attached mechanically against its substrate.
The electrolyte consists of concentrated sulfuric acid. Therefore, the weld between the connecting nickel lead and the tantalum lead from the sintered core is placed outside the can. The lead must not be bent inside the weld. Otherwise, the risk is high that the seal is damaged and sulfuric acid leaks out.
The sulfuric acid has a freezing point of approximately –64 °C. Its liquid state may sometimes give the capacitance a certain position dependency. This dependency is often avoided by giving the sulfuric acid a gelatinous consistency. The gelled electrolyte also is favourable with respect to the leakage risk.
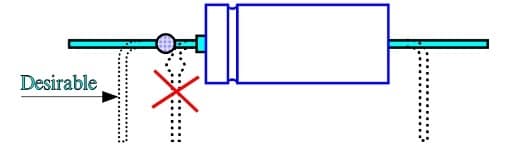
Figure 29. Bending rule for wet tantalum electrolytic.
The sintered wet electrolytic still is manufactured in a variant with silver can. The risk, however, of silver dendrite formation that causes a short-circuit is relatively high and the tantalum case is the preferred design. The conventional tantalum cathode sleeve design as per MIL-C-39006 style (Fig 27. left) is limited by CV, but it can stand a reverse voltage of 3 V up to 85 °C. The proprietary cathode design type as per DLA 93026 is offering significantly higher capacitance range and energy density but no reverse voltage is allowed.
The following series of diagrams are applicable to both Ag and Ta case styles if nothing else is said.
Properties
As with other wet electrolytic capacitors also, the wet tantalum has a strong temperature dependence on capacitance. It depends on the rather high resistivity of the electrolyte, especially at lower temperatures. This resistivity is, compared to solid electrolytes, high also at room temperatures which in turn is reflected on the presented frequency dependence measured at normal conditions. Figures 30. and 31.
Capacitance versus temperature
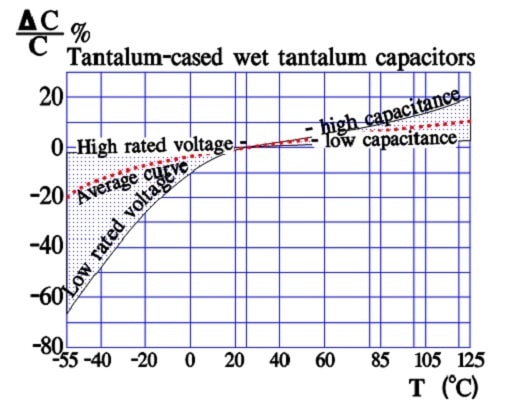
Figure 30. Typical curves for capacitance versus temperature in wet sintered tantalum capacitors
Capacitance versus frequency
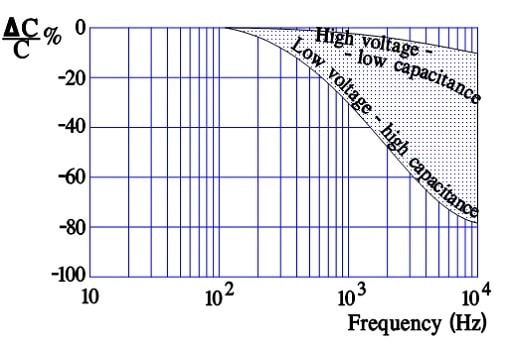
Figure 31. Typical curves for capacitance versus frequency in wet tantalum capacitors
The temperature dependence of silver-cased wet tantalum capacitors has a slightly broader curve range for low-rated voltage and high capacitance values.
Note in Figures 30. and 31. that “high rated voltage – low capacitance” constitutes one of the limits of the curve range and “low rated voltage – high capacitance” is the other limit.
Fine powder technology is a condition for low – and high-capacitance styles. The high porosity of the pellet, however, increases the ESR of the electrolyte due to the electrolyte-filled ducts that are becoming longer and finer and more resistive. The capacitance elements farthest in the pellet consequently require longer time constants for charging. When the frequency increases, these elements become ineffective, i.e., the capacitance decreases with frequency (Figure 31.).
The curve range in Figure 31. demonstrates a greater temperature dependence at low temperatures where the viscosity and resistivity of the electrolyte increase, the higher the pellet porosity is. Consequently, at lower temperatures, the upper limit of the curve range belongs to high capacitance designs.
ESR in silver-cased tantalums differs slightly from tantalum-cased wet capacitors by having a broader curve range at higher temperatures.
ESR versus temperature
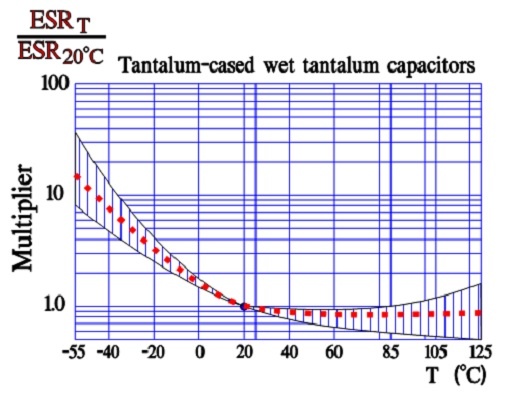
Figure 32. Normalized ESR versus temperature tantalum wet electrolytic capacitors. Reference: ESR at 20 °C
ESR versus frequency
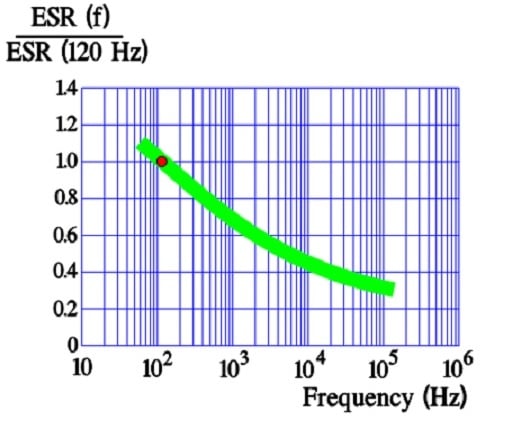
Figure 33. Typical curve of normalized ESR versus frequency on wet tantalum capacitors. Reference: ESR at 120 Hz.
The frequency dependence of ESR is presented in a normalized form, i.e., as a ratio between the ESR at different frequencies and at 120 Hz. Compared to solid MnO2 tantalums the frequency dependence of the ESR in wet tantalum capacitors is smaller and tends to level out at higher frequencies.
DCL versus temperature
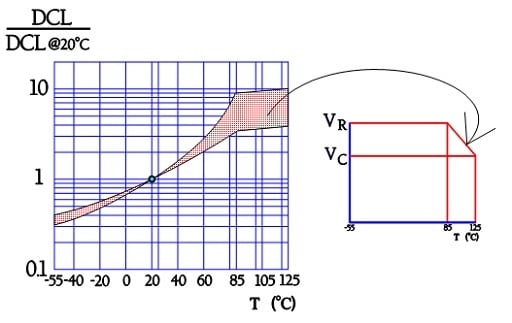
Figure 34. Normalized leakage current (DCL) versus temperature on wet tantalum capacitors. Reference: DCL at 20 °C
DCL versus applied voltage

Figure 35. Typical curves for normalized leakage current (DCL) on wet tantalum capacitors versus voltage in per cent of VR
The peculiar break in the leakage current curve depends on the derating that takes effect at 85 °C and stops further leakage current increase despite the in-creasing temperature.
Reference: DCL at 100%VR.
The leakage current follows an almost linear dependence of the applied voltage – which should be the case if the dielectric acted as a pure resistor. The dielectric, however, is no pure resistor but a complicated oxide formation that, together with the electrolyte and the base metal, creates a valve function. Therefore the curve bends slightly downwards at lower voltages. Compared with solid tantalum, this characteristic is even more pronounced, which has to do with the much larger margins to the formation voltage.
Failure modes
The most common failure mode is the leakage of electrolytes through damaged glass-to-metal seals. The failure can be provoked by careless lead bending and, not the least, by temperature changes. It’s advisable to ensure the manufacturer’s products can withstand temperature changes.
Other wet electrolyte case sizes and styles
Surface-Mount
As the whole electronic component industry moved towards surface-mount technology, the wet tantalum capacitor remained as an axial leaded device. The first early attempt to offer a surface-mountable wet tantalum capacitor was the Arcotronics SMTH. This was achieved by attaching special preformed SMD mounting tabs or terminations to the existing or standard axial tantalum case design. They could be manually soldered to the PCB using the tabs (see Figure 36.).
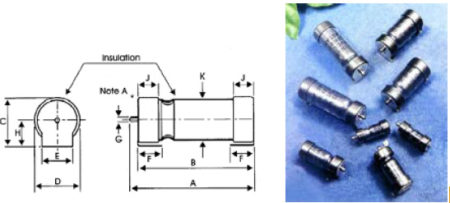
Figure 36. – SMTH SMD wet tantalum capacitor design drawing and photographs; source: Arcotronics
The next generation of surface-mountable wet tantalums was the M35 series, developed by Vishay. The M35 features a moulded style with terminations. The design incorporates a complete T1 axial all-tantalum wet tantalum capacitor, which is welded to a lead frame, and then moulded (see Figure 37.).
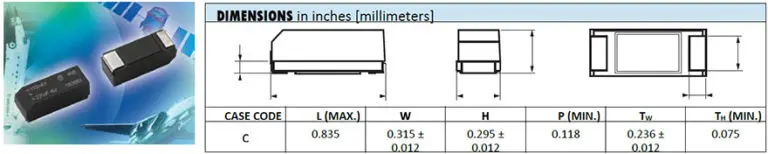
Figure 37. – M35 SMD wet tantalum capacitor series photo, drawing, and dimensions; source: Vishay
The latest addition by Vishay is the T22 series which is the first “true” SMD wet tantalum capacitor. It has a hermetically sealed rectangular tantalum case with terminations and is available in either bulk or a reel pack option (see Figure 38. below).
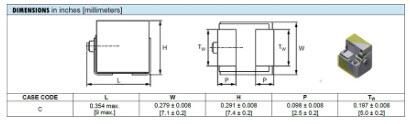
Figure 38. – T22 wet tantalum capacitor cross-section, dimensions and photo (right); source: Vishay
High Energy
Several axial wet tantalum capacitors are often connected in parallel and/or series for high energy or bulk power applications. While customers can do this on their printed circuit board (PCB), the capacitor manufacturer can also provide a pre-assembled module or array of capacitors. The original arrays were made of silver case capacitors, and there was actually a full MIL-PRF-3965 specification.
While these arrays are still available today, custom assemblies made from tantalum case wet tantalum capacitors are available from more vendors, such as Vishay, AVX, and Exxelia. An example is a module that consists of wet tantalum case T1 capacitors in Figure 39.
Wide variety of case materials and configurations to meet customer-specified performance requirements and are available with many termination options. Capacitor assemblies include balancing resistors, diodes, and other components to meet customer needs.
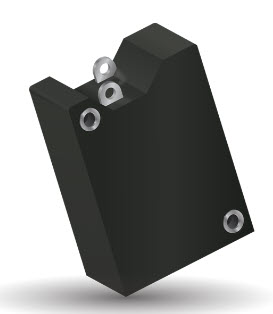
Figure 39. wet tantalum capacitors module
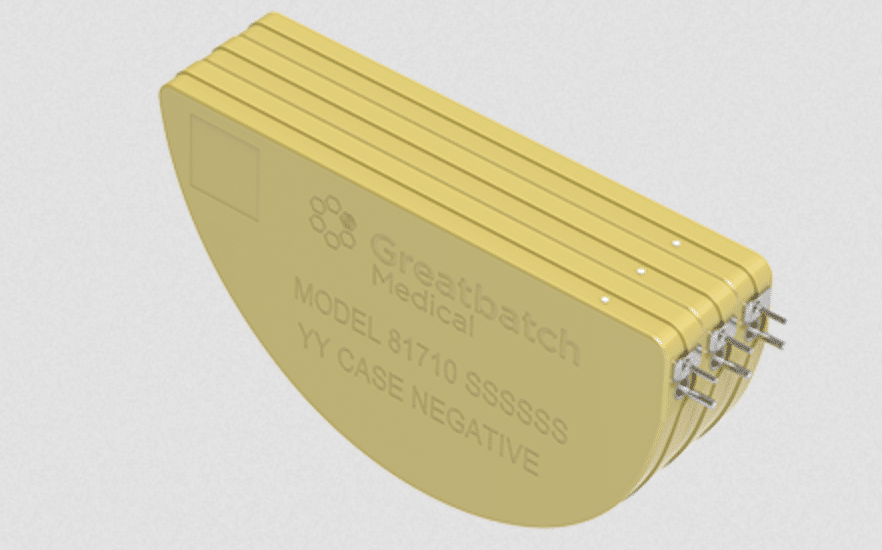
Figure 40. medical implantable tantalum wet capacitors for implantable defibrillator; source: Integer Greatbatch
In some high energy or bulk power applications, the best solution may be the relatively new high energy, sometimes termed “hybrid,” wet tantalum capacitors. These capacitors utilize a tantalum anode and tantalum case but need a hybrid cathode made by depositing a material such as ruthenium or palladium on a small piece of tantalum foil. These large case-size wet tantalums reach capacitance values of over 72,000μF at 25V and are used in energy hold-up and pulse power applications.
Very specialized designs, made with titanium cases, a special electrolyte, and rated for high voltages, have been used in medical implantable ICD applications for over 20 years. An individual ICD capacitor may provide 400μF at 250V in the relatively stable environment of the human body at an ambient temperature of 37ºC. See Figure 40.
Military versions of the high energy “hybrid” capacitors were developed in various configurations with one or multiple anodes in a cylindrical/rounded square case with radial terminations, such as illustrated in examples in Figure 41-43. below.
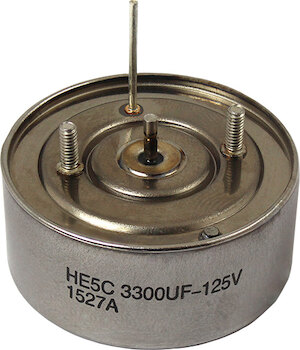
Figure 41. Vishay HE5 tantalum wet high energy capacitor
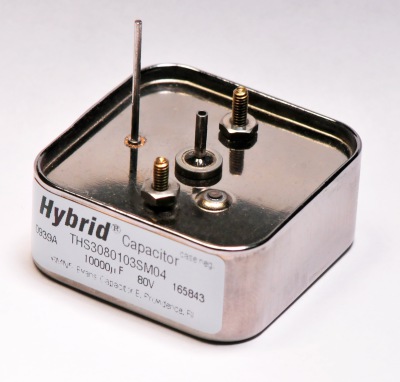
Figure 42. Evans THS hybrid wet tantalum capacitor
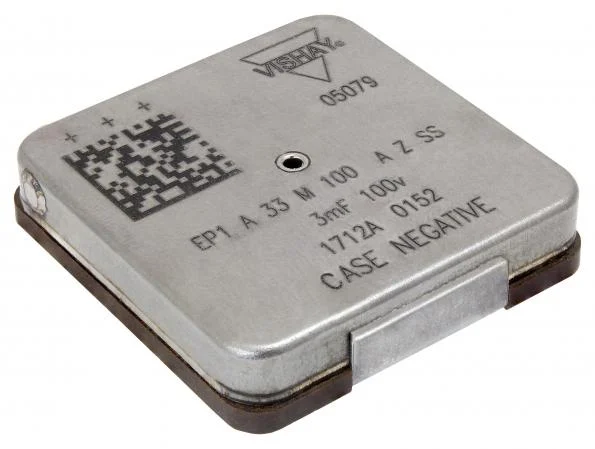
Figure 43. Vishay EP1 wet tantalum high energy capacitor
Source: Passive Components
- Managing EEE components for LEO and lower cost space missions - December 17, 2024
- Filtering Characteristics of Parallel-Connected Fixed Capacitors in LCC-HVDC - November 21, 2024
- ALTER SPACE TEST CENTER: testing approaches for New Space - September 30, 2024
0 comments on Tantalum and Niobium Capacitors