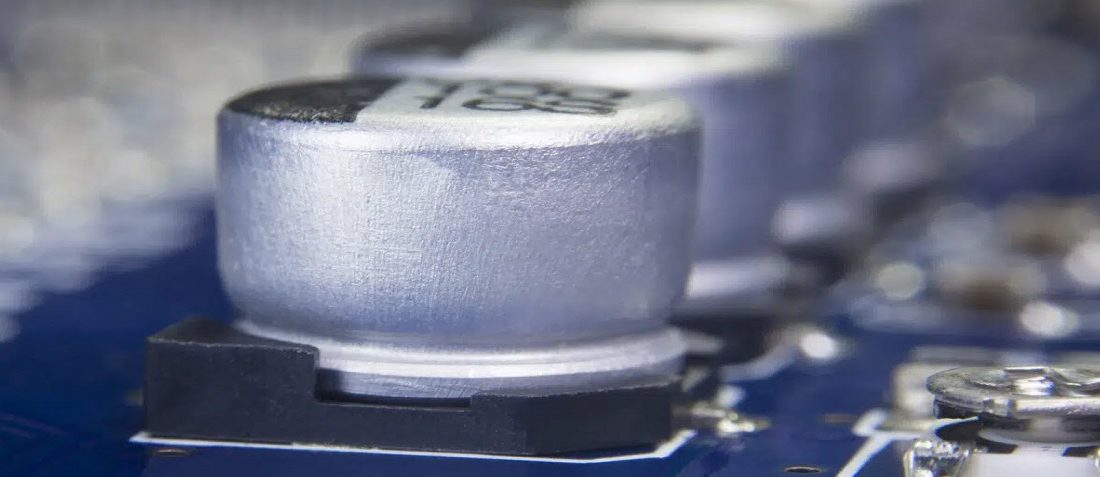
Aluminum Electrolytic Capacitors
- Posted by doEEEt Media Group
- On May 11, 2023
- 0
This article describes aluminum electrolytic capacitors’ types, features, characteristics and behaviour.
The primary strength of aluminium electrolytic capacitors is their ability to provide a large capacitance value in a small package and do so relatively cheaply.
Additionally, they tend to have good self-healing characteristics; when a localized weak spot in the aluminum oxide dielectric layer develops, the increased leakage current flow through the weak point in the dielectric causes a chemical reaction similar to that used during the initial formation of the dielectric layer, resulting in a thickening of the dielectric at the weak point, and a consequent reduction in leakage current.
Construction, Features and Manufacturing Process of Aluminum Capacitors
The shortcomings of aluminum capacitors are mostly related to
- the chemically-reactive nature of the materials used in their construction
- the conductive properties of the electrolyte solutions
- the volatility of liquid electrolytes.
The chemically reactive nature of the materials used in aluminum capacitors is problematic on two points: the dielectric layer’s stability and the device’s long-term mechanical integrity. Since the aluminum oxide dielectric layer in these devices is formed through an electrochemical process, it can also be eroded by an electrochemical process simply by reversing the applied voltage. This is why most aluminum capacitors are polarized; the application of voltage with the wrong polarity causes rapid erosion & thinning of the dielectric, resulting in high leakage current and excessive internal heating.
From a mechanical integrity standpoint, mixing a highly reactive metal (aluminum) with a corrosive electrolyte solution is a delicate proposition; errors in electrolyte composition can result in premature failure, as evidenced by the “capacitor plague” of the early 2000’s.
Another shortcoming of aluminum electrolytic capacitors is the fact that the electrolytes used aren’t particularly efficient conductors because conduction in electrolyte solutions is achieved through ionic rather than electronic conduction; instead of loose electrons moving between atoms serving as the charge carriers, ions (atoms or small groups thereof that have a charge due to a surplus or deficit of electrons) are moving about through the solution. Since ions are bulkier than electrons, they don’t move as easily and hence ionic conduction generally tends to be a higher-resistance proposition than electronic conduction. The extent to which this is the case is influenced significantly by temperature; the lower the temperature, the more difficult it is for ions in an electrolyte solution to move about through the solution, translating into a higher resistance. Thus, electrolytic capacitors tend to have a relatively high ESR that exhibits a strong inverse correlation with temperature.
The third major downside to aluminum capacitors (except the solid polymer types) is that the liquid electrolyte solutions tend to evaporate over time, eventually being lost to the atmosphere by diffusion through the rubber sealing plug, leaks in safety vent structures, or similar phenomena.
There are more types of aluminum electrolytic capacitors construction and termination styles:
- SMDs (V-chip) for surface mounting on printed circuit boards or substrates
- Radial lead terminals (single-ended) for vertical mounting on printed circuit boards
- Axial lead terminals for horizontal through-hole mounting on printed circuit boards
- Radial pin terminals (snap-in) for power applications
- Press-fit terminals
- Large screw terminals for power applications
The most common styles are wound foil capacitors packaged in aluminum cans as led or SMD termination styles. See Figures 1. and 2.
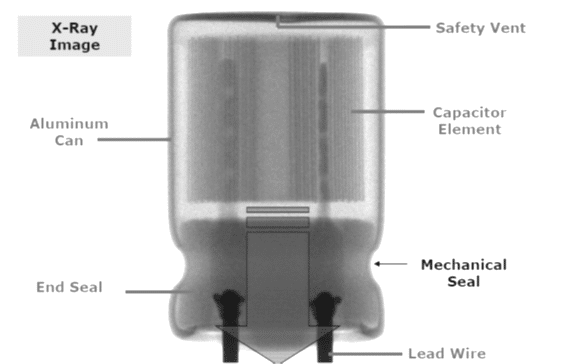
Figure 1. X-Ray image of aluminum can wet electrolyte leaded capacitors
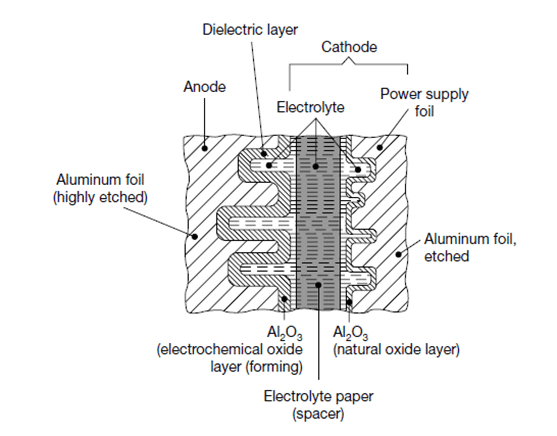
Figure 2 aluminum electrolytic capacitor structure drawing
Electrolyte can be wet, gel (TCNQ salt), solid (conductive polymer) or hybrid (combining wet and conductive polymer) based:
- 4 ~ > 500V
- high ESR
- poor temp performance
- dry out, cap decrease with life
- low cost
- 2.5 ~ 100V
- low ESR, high ripple current
- stable high and low temperatures
- leakage current stability issues
- higher sensitivity to humidity
- higher cost
- Up to 125V
- similar ESR as polymer capacitor
- more stable then liquid type
- better leakage current stability vs polymer cap
- higher cost comparing to wet
Panasonic, one of the suppliers of all aluminum and tantalum polymer capacitor technologies, provide a comparison of its technologies as follows:
- OS-CON is a TCNQ salt electrolyte
- Hybrid combines wet and polymer electrolytes
- SP-Cap is a solid polymer chip capacitor
- POSCAP is a tantalum polymer capacitor
The following chart in Figure 3. demonstrates the lifetime with temperature comparison of wet vs polymer vs hybrid aluminum capacitors that can be helpful for a specific application selection guide.
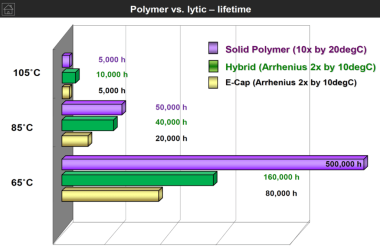
Figure 3. Polymer versus Electrolytic versus Hybrid aluminum capacitors lifetime; Source: Panasonic
DCL and balancing
Aluminum electrolytic capacitors’ leakage current and balancing is explained in more detail in the paper below:
DCL of Aluminum Electrolytic Capacitors – by Dr Arne Albertsen from Jianghai Europe Electronic Components GmbH
Manufacturing Process:
The production process starts with mother rolls. First, the etched, roughened and pre-formed anode foil on the mother roll, as well as the spacer paper, and the cathode foil, are cut to the required width.
The foils are fed to an automatic winder, which makes a wound section in a consecutive operation involving three sequential steps: terminal welding, winding, and length cutting. In the next production step, the wound section fixed at the lead out terminals is soaked with electrolyte under vacuum impregnation.
The impregnated winding is then built into an aluminum case, provided with a rubber sealing disc, and mechanically tightly sealed by curling. Thereafter, the capacitor is provided with an insulating shrink sleeve film. This optically ready capacitor is then contacted at a rated voltage in a high-temperature post-forming device for healing all the dielectric defects resulting from the cutting and winding procedure.
After post-forming, a 100% final measurement of capacitance, leakage current, and impedance occurs. Taping closes the manufacturing process; the capacitors are ready for delivery.
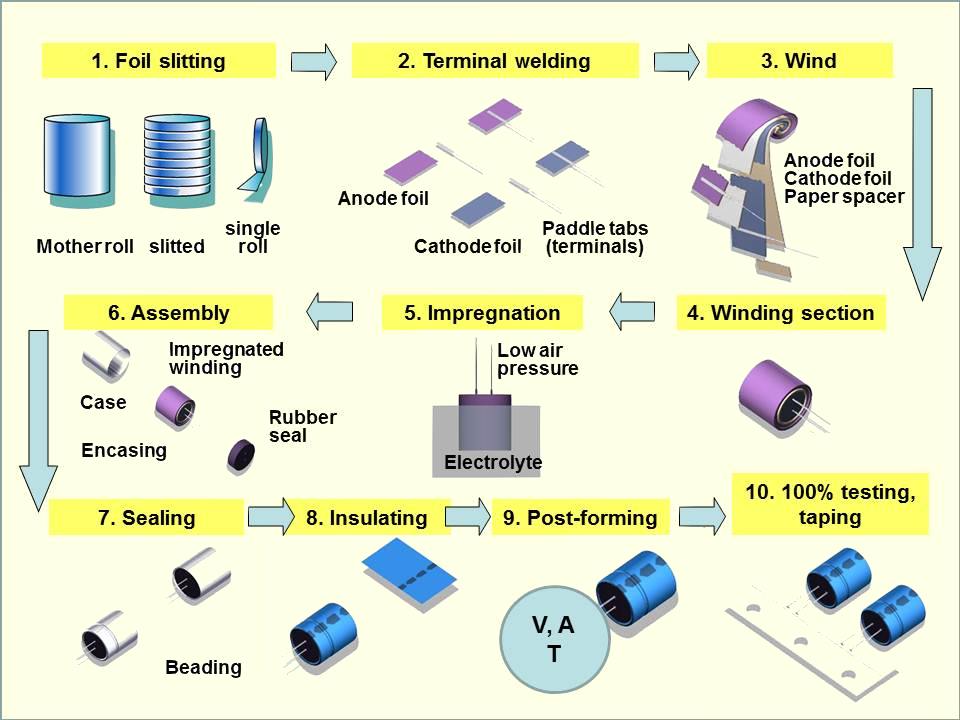
Figure 4. The manufacturing process of aluminum electrolytic capacitors. source: Wikipedia
Wet Aluminum Electrolytic Capacitors
Introduction
Wet aluminum (Al) electrolytic capacitors continuously generate hydrogen gas under operation while storing it with a small residual charge. That hydrogen must be able to diffuse and exit the package, or it will rupture. Thus conventional wet Al electrolytes must not be hermetically sealed. The diffusion occurs through the non-hermetic lid that usually has a rubber packing in the pressure seal against the can edge.
Larger size cans are also supplied with a safety vent that provides relief in the instance of very critical gas generation. There are also special, high-reliability hermetic Al electrolytic available on the market based on improvements in electrolytes and gas-absorbing compounds.
In catalogue sheets for different electrolytic types, it’s usual to show impedance versus frequency at different temperatures. The axes are given a logarithmic scale and look in principle like the one in the following Figure 5. where the ESR at several temperatures can also be read.
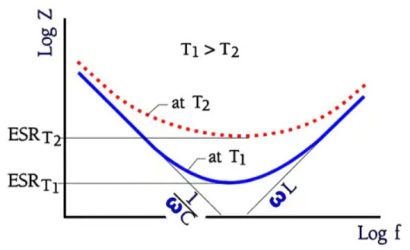
Figure 5. Aluminum electrolytic capacitor Impedance versus frequency at two temperatures T1 and T2
The capacitance stability versus time and temperature is comparatively poor. Tolerances must be adjusted from ±20 for the best professional types to –20/ +80% for some commercial makes. Rated voltages exist from 3 to above 500 V DC.
The maximum permissible reverse voltage is:
- 0.5 V for VR ≤ 7 V
- 1 V for VR > 7 V
The reverse voltage figures apply up to the rated temperature. Then a proper derating is recommended. Losses often are large and strongly temperature and frequency dependent. Figure 5. shows how the impedance versus frequency curve at increasing frequencies approaches and finally reaches the ESR contribution at temperature-dependent levels. This occurs somewhere between 1 to 100 kHz, depending on capacitance size and temperature.
Recent examples of new solid-polymer or hybrid electrolytes with improved electrical conductivity are present on the market. Nevertheless, the conventional wet Al electrolytic capacitors are one of the lowest-cost capacitor technologies on the market and are still under high volume use or in higher voltage applications where there is no polymer/hybrid electrolyte alternatives.
Examples of some typical applications of wet Al electrolytes are as follows: smoothing, filtering in Switched Mode Power Supplies (SMPS; low ESR necessary), coupling/decoupling, energy storage, timing and delay circuits with large time constants.
Properties
Figure 6. shows a strong capacitance deviation at lower temperatures. The increasing resistivity of the electrolyte causes it. Note that the temperature ranges vary, and the temperature dependence of corresponding electrolytes also varies. Some types can withstand –55/+150 °C, others only –25/+85. The most common temperature ranges are –40/+85 and -40/+105 °C.
Capacitance versus temperature
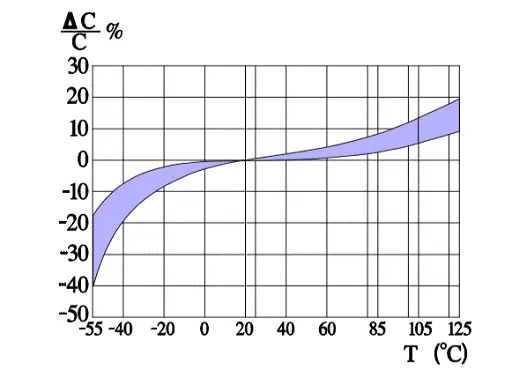
Figure 6. Typical curve range for capacitance versus temperature T for wet aluminum electrolytic capacitors
Capacitance versus frequency
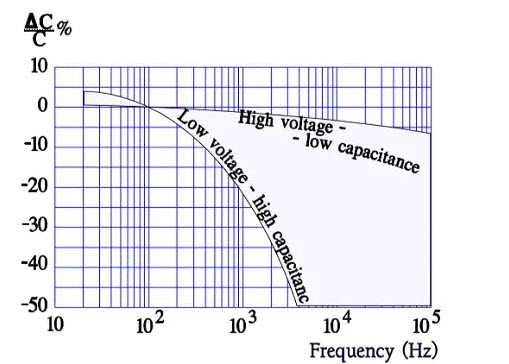
Figure 7. Typical curve range for capacitance versus frequency of wet aluminum electrolytic capacitors
Note in Figure 7. how the low voltage – high capacitance, characterized by large foil surfaces and finer etching, demonstrates a higher frequency dependence while the opposite decrease this dependence.
Tan δ versus temperature
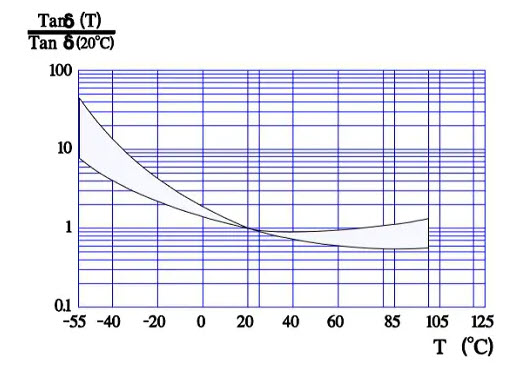
Figure 8. Typical curve range for normalized Tanδ versus Temperature for wet aluminum electrolytic capacitors
Figure 8. shows the normalized Tan δ value, i.e., the multiplier for Tan δ compared with the reference value at 20 °C. Thus, according to the curve, in the worst case, Tan δ 55°C is approximately 50 times larger than Tan δ+20°C.
According to the formula Tan δ = ESR x ωCs, which means that at a fixed frequency, the ESR versus temperature curve will have the same appearance as the one of Tan δ (Figure 9.).
ESR versus temperature
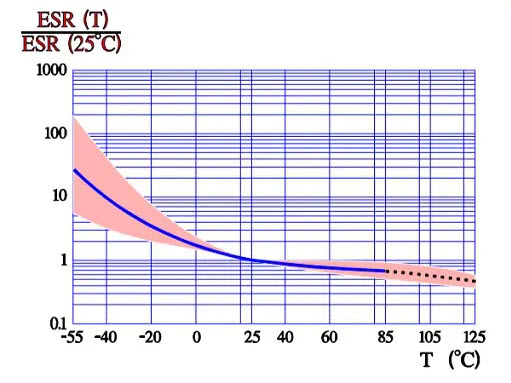
Figure 9. Normalized ESR range versus temperature T in wet aluminum electrolytic capacitors. Reference value at 25°C
ESR versus frequency
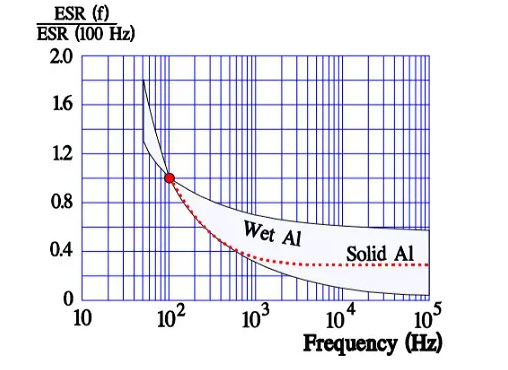
Figure 10. Typical curve range for normalized ESR versus frequency for wet aluminum electrolytic capacitors. Reference at 100 Hz
In Figure 10. the ESR versus frequency is shown as a ratio between the 100 Hz value and those at other frequencies (a multiplier of the reference value). It applies at room ambient temperatures and above and will differ slightly at lower temperatures. The strong slope of the curve range at lower frequencies depends mainly on the oxide losses. Just for caparison, a typical curve also for solid Al is shown.
ESR and ripple currents
In filter applications, the capacitors will conduct considerable AC currents caused by superimposed ripple. The ripple current creates heat in the capacitor ESR. Therefore a maximum ripple current IR-max is specified at reference conditions: 100 or 120 Hz and at some higher temperature, for example, 85 °C or the upper category temperature Tuc (Figure 6.). With reference data in the data sheets as a starting point, it is then possible to convert these values to operational condition values.
The maximum ripple current is specified to create a generated average core temperature rise of 10 °C above the allowable maximum ambient temperature. This margin, however, varies strongly with the can size and manufacturer. Concerning proper derating, this margin should not be utilized. When the ripple current frequency rises, the ripple current capability increases. That depends on a corresponding decrease of the ESR (Figure 10.) that has a negative TC, mainly caused by the electrolyte, and this will consequently lead to a decreasing ESR when the temperature rises (for example, at higher ripple currents) (Figure 9.). If we, for example, specify an IR-max at 100 Hz and permit 1.4 x IR-max at 10 kHz, this is possible just because ESR10kHz ≈ ½ESR100Hz. For (1.4 x IR-max )2 x ½ ESR100Hz ≈ 2 x ½ x (IR-max )2 x ESR100Hz = (IR-max)2 x ESR100Hz. But please check the manufacturer’s data sheets. There are differences between both styles and manufacturers.
Leakage current
Also, the important parameter leakage current varies with the environmental conditions. When temperature increases from the lower to the upper limit of the temperature range, leakage current rises approximately two orders of magnitude (Figure 11.). The next figure shows the ratio of DCL versus applied DC voltage and DCL at 100% VR.
Leakage current versus temperature
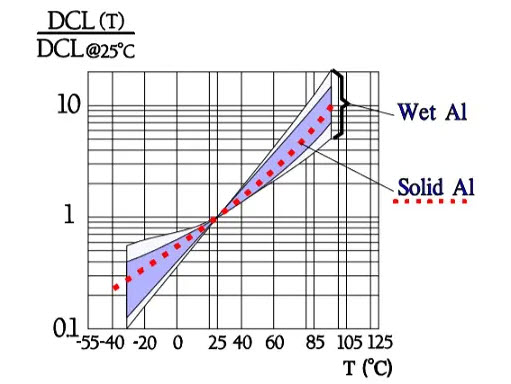
Figure 11. Normalized typical curve range for DCL leakage current versus temperature for aluminum electrolytic capacitors. Reference DCL at 25 °C
Leakage current versus DC voltage
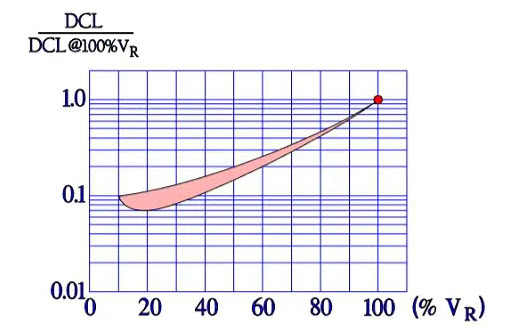
Figure 12. Normalized typical curve range for DC Leakage versus DC voltage for wet aluminum electrolytic capacitors.
Heat generation
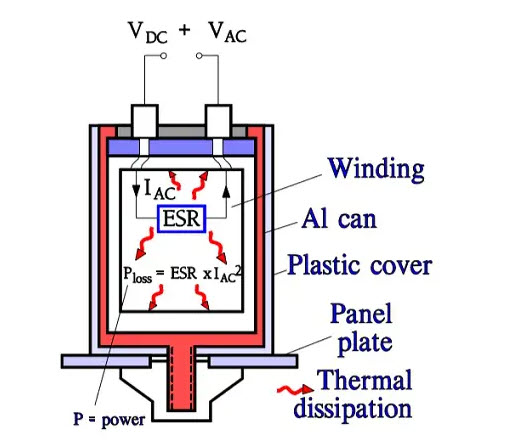
Figure 13. Thermal dissipation in large aluminum electrolytic capacitors with internal heat generation
Aluminum electrolytic also are manufactured with high CV products in correspondingly large cans. If they are loaded with a superimposed ripple voltage, heat generation from the power ESR x IAC2 might be troublesome. The rise of temperature will be largest in the centre of the winding, i.e. the capacitor Hot Spot, and must not exceed certain values. Therefore a maximum AC value is specified at 100 or 120 Hz and sometimes at 20, 40 or 100 kHz. Furthermore, it’s usual to state the maximum ESR at these frequencies. A schematic of the heat generation principle is shown in Figure 13. The components of ESR are shown in Figure 14.
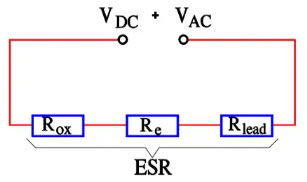
Figure 14. Schematic of ESR components of aluminum electrolytic capacitors
- Rox = resistance in the dielectric oxide layer
- Re = resistance in the electrolyte and paper separator
- Rlead = resistance in electrical conduction paths.
Above all, the dielectric oxide losses are frequency dependent and cause the general appearance of the ESR curve shown in Figure 10. In more detail, the frequency dependence can be illustrated as in Figure 15 where one can note how the oxide losses, on the whole, end above 1.5 kHz.
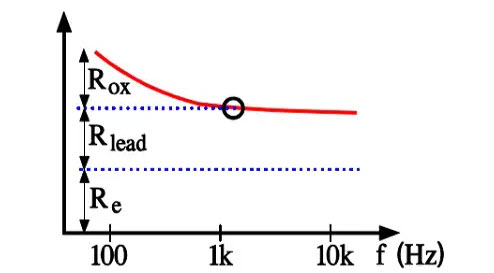
Figure 15. Frequency dependence of the oxide losses in aluminum electrolytic capacitors
There are for high-reliability styles information about their thermal capacitance and resistance. If we know the can and ambient temperatures, then the Hot Spot temperature of the capacitor can be calculated more thoroughly. A less accurate but more simple calculation says that
Thsp – Ty » Ty – Ta⋅⋅⋅⋅⋅⋅⋅⋅⋅⋅⋅⋅⋅⋅⋅⋅⋅⋅⋅⋅⋅⋅⋅⋅⋅⋅⋅⋅⋅⋅⋅⋅⋅⋅⋅⋅⋅⋅⋅⋅⋅⋅⋅⋅⋅⋅⋅⋅⋅[1]
Where:
- Thsp = Hot Spot temperature
- Ty = surface temperature of the can
- Ta = ambient temperature (Figure C3-35)
Life calculation
The Life (L0) for a wet Al electrolytic is stated in the catalogue sheets as the number of hours at the maximum temperature, Tuc. If, for example, the stated L0 = 2000 h @ 85 °C, we get the Life at 35°C by means of the formula as

7 years
Thus the formula says that Life is doubled for a temperature decrease ∆T = 10 °C. We repeat that the formula is just a rule of thumb (Arrhenius relationship) and has no accurate calculation.
Mounting
Large can electrolytes with a safety vent should preferably be mounted in an upright or horizontal position, thus preventing electrolytic liquids from obstructing any gas diffusion.
Cleaning solvents
Penetrating contaminations, especially from halogen-based washing agents, may lead to a short circuit sooner or later. Exposed to an electric field, they will be converted electrochemically to free chlorides that will cause corrosion, a subsequent increase in leakage current, and a short circuit. Because destruction occurs only when the capacitor is electrically operated, failures occur only in the field and after some time. To avoid a suction effect during the washing, one shall ensure that the component has a lower temperature than the washing bath.
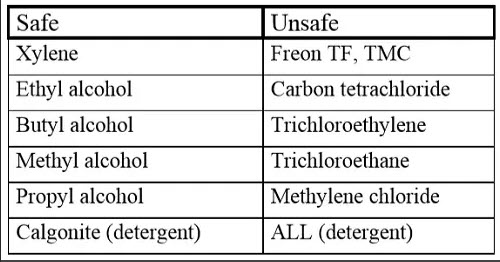
Table 1. Examples of cleaning solvents
Failure modes
The most common failure is the drying escape of the electrolyte. Drying first manifests itself as a high ESR, for it’s not only hydrogen gas that diffuses through the sealings to the lid of the aluminum electrolytic. The electrolytic liquid also diffuses through the non-hermetic lid. One leading manufacturer has solved this problem to a great extent by utilizing rubberized laminated paper washers instead of conventional rubber seals. However, diffusion and hydrogen development will take their tribute. Gradually the electrolyte dries up, and left will be only a kind of resistor.
Experiences indicate that an electrolyte loss of 20% increases the ESR by approximately 100%! The wet Al electrolytic capacitor is the one component type that has the most distinct life limitation. Beyond a certain time, the capacitor ceases to be a capacitor. At tests performed at the maximum temperature, one may get an idea of the diffusion rate and, thus, the life by weighing the part and calculating the electrolyte loss during the test. Wet Al electrolytes in chip design had some problems when they were first introduced on the market. There were examples of how certain types lost half of the electrolyte supply during the soldering process.
Now these problems certainly are overcome, but make sure the manufacturer is in command of the difficult manufacturing process of chip sealing. Open circuits caused by fatigue fractures in internal contact strips between winding and terminals during vibration/bump are not too unusual. Chloride contamination of foils from clammy hands or penetrating cleaning agents can cause high leakage currents and finally short-circuiting.
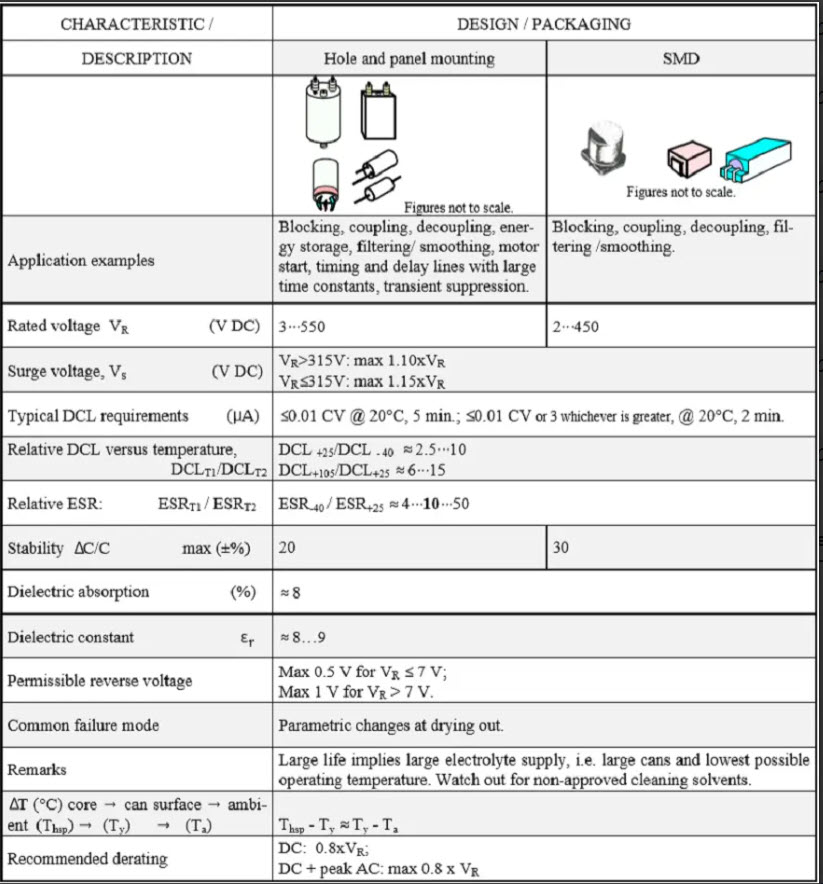
Table 2. WET ALUMINUM ELECTROLYTIC CAPACITORS CHARACTERISTICS
Solid Aluminum Electrolytic Capacitors, Polymer, Hybrid and TCNQ Salt
Solid Aluminum Electrolytic Capacitors with Conductive Polymer or TCNQ Salt
Polymer Electrolytic Capacitors
The most common variant of a solid electrolyte is conductive polymer electrolyte. The aluminum oxide on an etched and formed foil is covered with an electrically very conductive and doped polymer. The polymer can withstand temperatures up to +105 °C. The ESR of the capacitor is several ten powers better than that of the wet electrolytic types. Its temperature dependence is negligible, but the frequency dependence is more pronounced (Figure 16.).
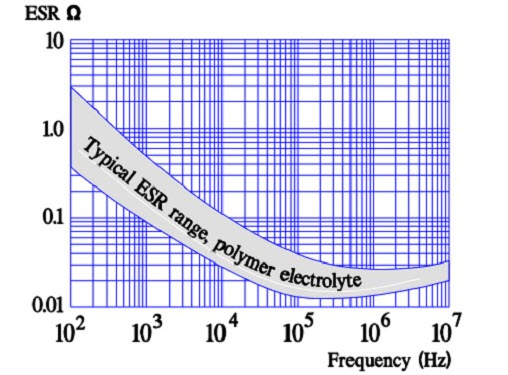
Figure 16. Typical ESR versus frequency in aluminum polymer capacitors.
The polymer electrolytic capacitor is manufactured in a can or a chip construction with an ESR range from 4.5mΩ to 70mΩ, voltage range from 2 V to 16 V and capacitance from μF to hundreds of μF. Recommended derating is to use ≤ 80% of VR. The capacitor must not be subjected to any reverse voltage. In case of a short-circuit, the local heat generation from the current in the failure spot will be so high that the polymer either will oxidize or will be de-doped and thus create an insulating “patch” over the failure area. Thus, the capacitor self-heals.
An advantageous chip design is the flat stacked type shown in Figure 17. It consists of stacked aluminum plates that have been etched and supplied with anodic oxidation. The tunnel-like structure is covered with a polymerized thin conductive film. Paint is added as a graphite layer between this film and the following silver, serving as a buffer between the polymer and the silver coating.
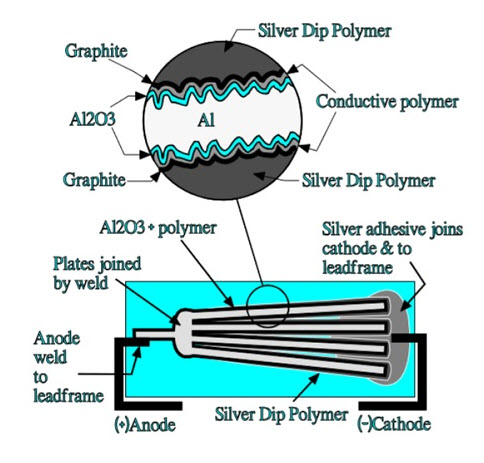
Figure 17. Schematic of SMD chip aluminum polymer capacitors.
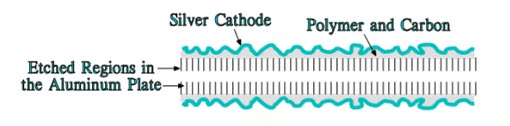
Figure 18. Schematic of an etched aluminum plate with additional cathode layers on aluminum polymer capacitors.
The aluminum plates are dipped in a silver epoxy that covers the polymer and graphite surface and fills up the spongy structure of the aluminum plates. The device is then molded in the same plastic epoxy as the one used in solid tantalum SMDs. This construction has excellent volumetric efficiency. There is a significant difference between this construction and that of a solid sintered tantalum capacitor.
In the latter case, the current has to pass “horizontally” through the MnO2 cover to charge the inner capacitive elements in the porous tantalum pellet. In the stacked aluminum construction, the current passes “vertically” through the silver, carbon and polymer layers to the inner capacitive elements in the etched tunnels as shown in Figure 18.
The vertical current paths mean a considerably reduced ESR. Such capacitors are capable of a single-digit ESR in the 100 kHz region.
The polymer capacitor is well established and has its greatest advantages in smoothing and noise reduction applications. For high-reliability applications, however, its capability in high-shock mechanical environments must be evaluated.
Hybrid Aluminum Electrolytic Capacitors
There also exists a hybrid version of the polymeric style. It has a construction of principle, as shown in Figure 19. Combining a liquid electrolyte and a polymer reduces the ESR, while the self-healing properties are retained due to the ion movability.
This type is growing presence on the market as Al can construction (SMD variant is also available) due to its improved robustness to IR reflow mounting conditions compared to Al pure polymer electrolyte solution.
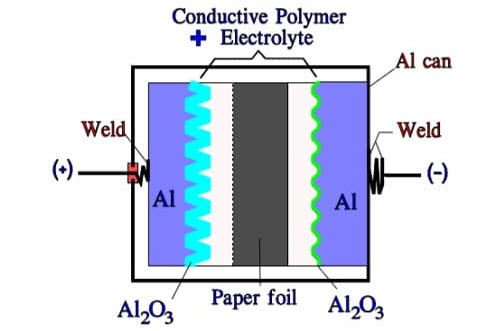
Figure 19. Schematic of a hybrid aluminum electrolytic capacitor with aluminum can and cathode foil.
TCNQ Salt Aluminum Electrolytic Capacitors
Like the polymer electrolyte, the semiconducting organic salt TCNQ has also been available on the market for several years under the trade name OSCON. The winding is impregnated at a relatively high temperature with melted salt that, at cooling, is transformed into a solid state. Its ESR is better than that of a wet electrolyte but somewhat poorer than that of a polymer electrolyte. The temperature dependence of the ESR is negligible, but its frequency dependence is more pronounced (Figure 20.). Note its similarity with the polymer electrolytic.
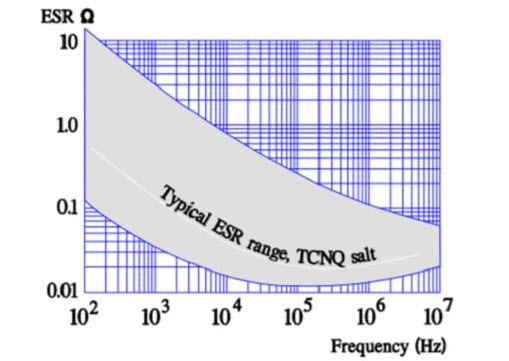
Figure 20. Typical ESR versus frequency in TCNQ OSCON aluminum electrolytic capacitors.
This capacitor is sensitive both to mechanical shocks and ESD. It can endure certain vibration environments. Immediately after soldering, it should not be subjected to handling due to the heat’s influence on the structural strength of the electrolyte/ dielectric system. The highest rated voltage should be derated linearly from 25 V at 85 °C to 20 V at 105 °C.
- Capacitance range 0.1⋅⋅⋅680 µF
- Temperature range -55/+105°C
- Maximum rated voltage 30 V DC
- ESR, frequency and temperature dependence ≈ of the polymer capacitor.
Self-healing may occur at short-circuiting. In the failure spot, the short-circuit current generates so much heat that the salt decomposes or disappears and thus creates a local insulation area. The capacitor must not be subjected to surge currents or any overvoltage. Prolonged load at high temperatures will lead to capacitance decrease or, in the worst case, to open mode. TCNQ salt may also present some risk to the environment and ROHS regulation due to the presence of cyanide salts. Thus polymer/hybrid types are the main growing aluminum electrolytic capacitor technologies at the present stage.
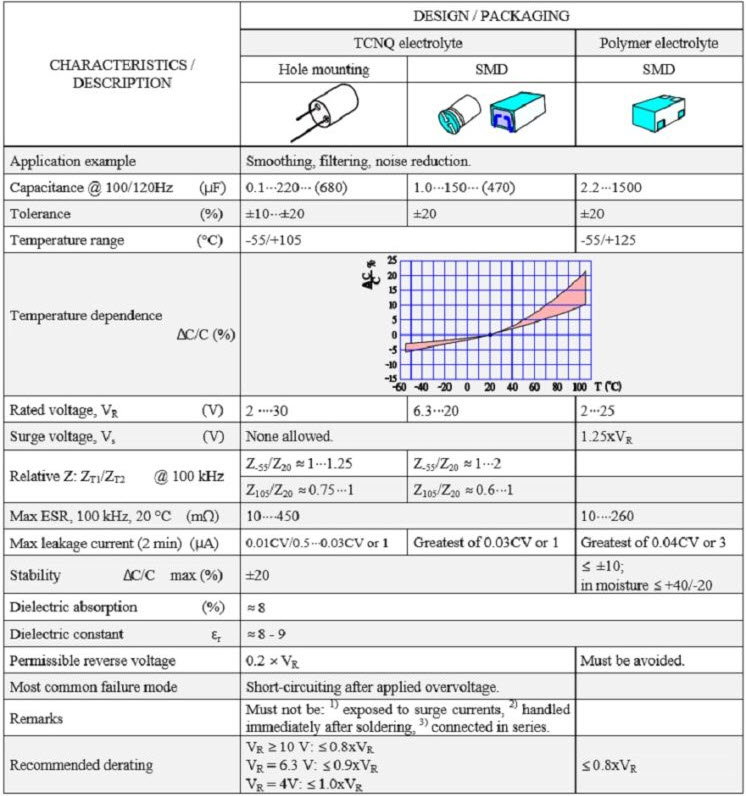
Table 3. SOLID ALUMINUM ELECTROLYTIC CAPACITORS, POLARIZED, with CONDUCTIVE POLYMER or TCNQ SALT
Solid Aluminum Electrolytic Capacitors (SAL) with Manganese Dioxide MnO2 (obsolete capacitor technology)
The SAL are aluminum electrolytic capacitors with anodic oxidized aluminum oxide as dielectric and the semiconducting solid manganese dioxide as electrolyte. They are made of etched and formed aluminum anodes, which are folded for the dipped pearl types or wound into a roll for the axial style. The solid manganese dioxide electrolyte is formed onto this roll in a pyrolytic process, similar to that for solid tantalum capacitors.
SAL-capacitors were developed and introduced in the market in the 1960s by Philips. Until December 30, 2015, it was a single-source product manufactured by Vishay.
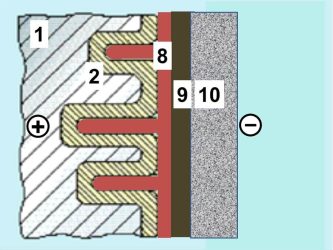
Figure 21. Principle cross section of a SAL solid aluminum electrolytic capacitors with solid manganese oxide electrolyte, graphite/silver cathode connection, 1: Anode, 2: Al2O3, 8: MnO2, 9: graphite, 10: silver; source: Vishay
As of December 31, 2015 the SAL are end-of-life and have ceased production. Nevertheless, we still list the technology features here for comparison and overview.
Introduction
The electrolyte is a solid one. Thus, life is theoretically unlimited and independent of drying effects and leakage currents. Storage has no impairing effect on the dielectric. The stability is much better than that of wet types. The capacitance tolerance is ±20%. The price is high.… Compared to solid tantalum, the price for solid aluminum (Al) electrolytes of the same CV product is between two and three times higher. The solid electrolyte doesn’t penetrate the pores of the highly etched aluminum foil equally well as a wet one. Its conductivity, however, is between one and two orders of magnitude larger than that of the wet electrolyte, and its temperature dependence is smaller.
Thus, the solid Al electrolytic is relatively insensitive to the pore effect. In wet Al, electrolytes create a deep network of series and parallel resistances, as shown in the electrolytic capacitors overview. The capacitor can’t stand any breakdowns. This requires large margins between the forming and rated voltages. The forming rate usually is 2 to 3 times, and the rated voltage maximum is 40 V DC. Unlike solid tantalum, the capacitor is relatively insensitive to pulse loads which, among other things, depends on its higher ESR that reduces the need for an additional circuit impedance.
Nevertheless, high pulse currents can initiate a fire, like in solid tantalums. There were two construction styles on the market, the epoxy encapsulated radial types for a maximum 175 °C and the axial aluminum can type designed for maximum 200 °C. The radial lead design has a stacked aluminum plate construction which renders a very low ESL (equivalent series inductance). It ranged from 9 to 20 nH. The cylindrical can have a winding of anode and cathode foils plus glass fibre spacers. The reliability of the cylindrical can types is high. Of these, there are designs with epoxy fillings intended for extreme acceleration and shock stress.
Properties
Both the radial and the axial lead types are represented in Figure 22.
In comparison with wet aluminum electrolytes (Figure 9.) ESR of the solid ones have a less pronounced temperature dependence as shown in Figure 23.
Capacitance versus temperature
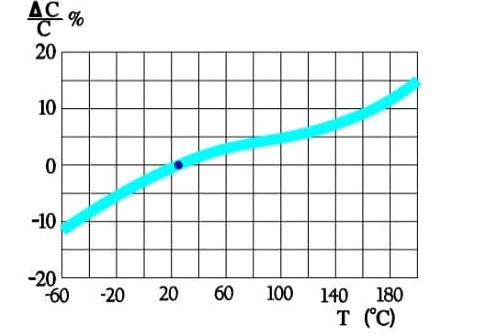
Figure 22. Typical temperature dependence in solid aluminum electrolytic capacitors
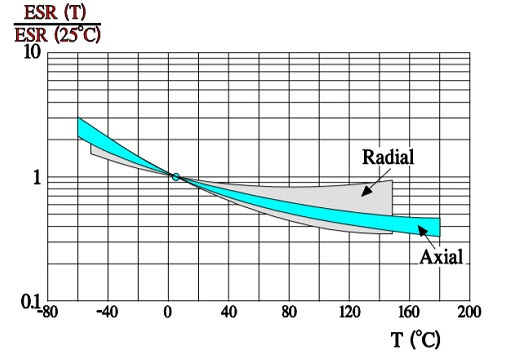
Figure 23. Normalized ESR versus ambient temperature in solid aluminum electrolytic capacitors with different temperature ranges. Reference ESR at 25 °C
ESR versus frequency Typical normalized diagrams are shown in Figures 24. and 25. The former concerns radial lead designs with lower ESL and higher operating frequency ranges.
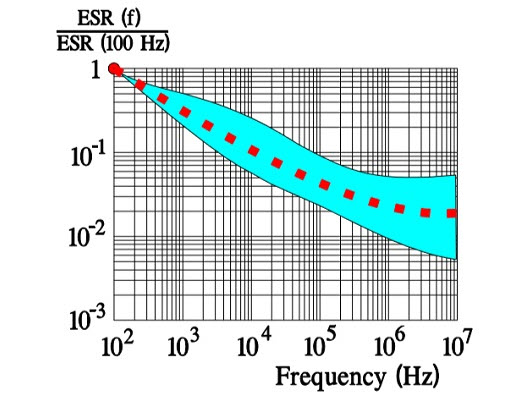
Figure 24. Normalized ESR versus frequency in solid aluminum electrolytic capacitors with radial leads. Reference ESR at 100 H
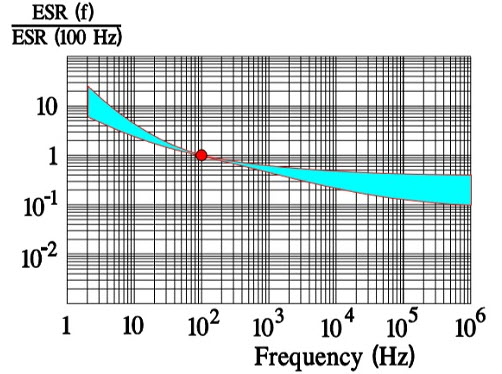
Figure 25. Normalized ESR versus frequency in solid aluminum electrolytic capacitors with axial leads. Reference ESR at 100 Hz
Note the lower frequency range of the following axial lead design. The steeper slope of the diagrams in the lower part of the frequency range depends on the oxide losses (Figure 25.).
Leakage current versus temperature
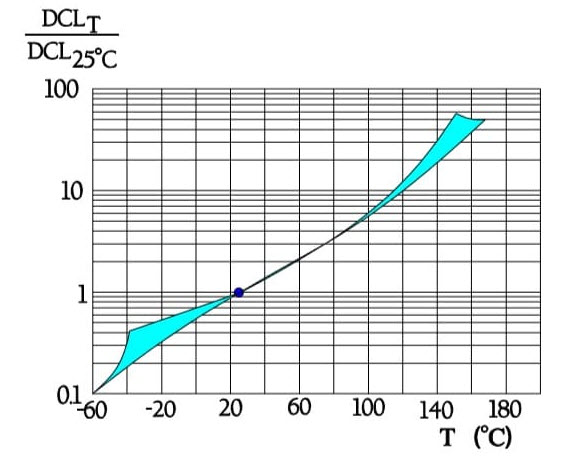
Figure 26. Normalized leakage is current versus temperature in solid aluminum electrolytic capacitors. DCL reference at 25 °C
Reverse voltage
The permissible reverse voltage is stated to be:
- @ ≤ 85°C max 0.3 x VR
- @ 85 → 125°C from max 0.3xVR → max 0.15xVR
Failure modes
Epoxy-dipped types with radial leads are sensitive to mechanical forces applied on the leads at mounting—inappropriate handling increases the leakage current.
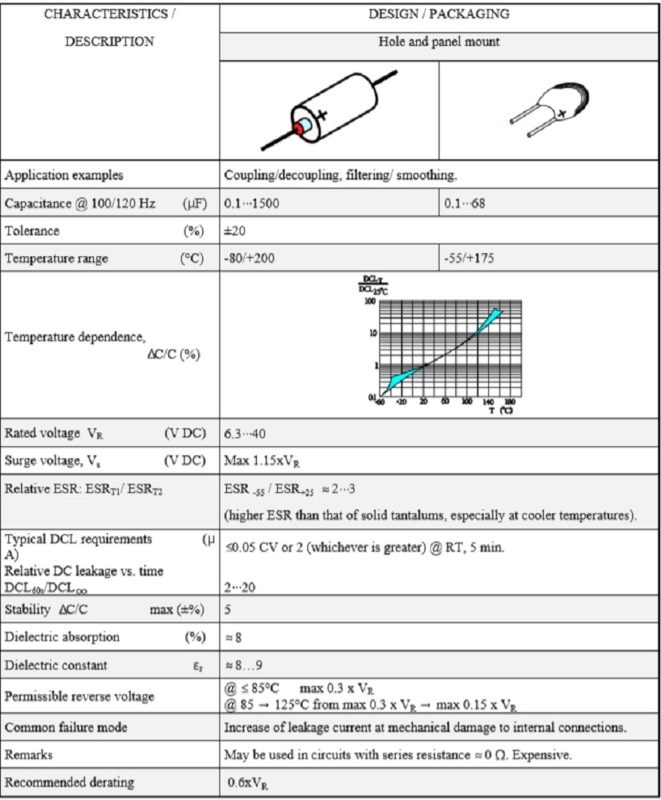
Table 4. SOLID ALUMINUM ELECTROLYTIC CAPACITORS, POLARIZED, WITH MANGANESE DIOXIDE
Source: Passive Blog Components
- ALTER SPACE TEST CENTER: testing approaches for New Space - September 30, 2024
- Failure Mechanism of Metallized Film Capacitors under DC Field Superimposed AC Harmonic - September 5, 2024
- Film Capacitor Technologies and Applications 2024 - September 5, 2024
0 comments on Aluminum Electrolytic Capacitors