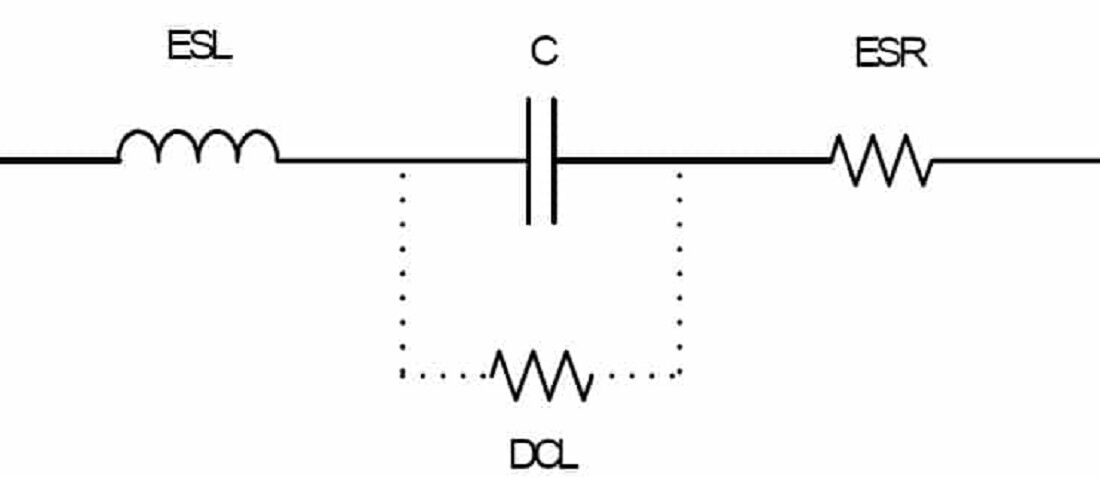
Leakage Current Characteristics of Capacitors – Case Study
- Posted by doEEEt Media Group
- On March 3, 2023
- 0
Capacitors, just like other electronic components, are constructed with imperfect materials. The imperfections and defects in these materials have significant effects on the electrical performance of capacitors. Some of the parameters determined by these defects and imperfections include impedance, dissipation factor, inductive reactance, equivalent series resistance, and leakage current. When designing an electronic circuit, it is necessary to consider the leakage current characteristics of capacitors.
DC leakage current is one of the key characteristics to consider when selecting a capacitor for your design. Other important parameters include working voltage, nominal capacitance, polarization, tolerance, and working temperature. Basic leakage current definitions and their reciprocal value – insulation resistance can be found in the following article here.
Leakage current and its effects on the performance of capacitors
A dielectric material separates the conductive plates of a capacitor. This material does not provide the perfect insulation and allows current to leak. The DC leakage current refers to this small current that flows through a capacitor when voltage is applied. The value of this current mainly depends on the applied voltage, capacitor temperature, and charging period.
The amount of leakage current varies from one type of capacitor to another, depending on the characteristics of the dielectric material and construction. Aluminium electrolytic capacitors have a large leakage current, while ceramic, foil, and plastic film capacitors have small leakage currents. A tiny leakage current is commonly called “insulation resistance”.
In electronic circuits, capacitors are used for a wide range of applications, including decoupling, filtering, and coupling applications. Some applications, such as power supply systems and amplifier coupling systems, demand capacitors with low leakage currents. Aluminium electrolytic capacitors and tantalum capacitors have high leakage currents and are generally unsuitable for such applications. Plastic and ceramic capacitors have lower leakage currents and are commonly used for coupling and storage applications.
Leakage Current vs. Insulation Resistance
The dielectric materials used in capacitors are not ideal insulators. A small DC current can flow or “leak” through the dielectric material for various reasons specific to each dielectric. As a result, when a capacitor is charged to a certain voltage, it will slowly lose its charge. As it loses its charge, the voltage between the capacitor’s electrodes will drop.
The leakage current (DCL) and the insulation resistance (IR) are in simple mathematical relation to each other:
R (IR) = V / I (DCL) or I (DCL) = V / R (IR)
Since the values are related, the terms leakage current and insulation resistance will vary depending on the dielectric type. Aluminum electrolytic capacitors have a relatively large leakage, thus called leakage current. Alternatively, plastic film or ceramic capacitors have a very small leakage current, so the effect is quantified as insulation resistance. See figure 1. overview of IR on most common capacitor dielectric types.
Generally, insulation resistance tends to decrease with higher values of capacitance. For practical reasons, the insulation resistance may be expressed in Megaohms at low capacitance values and in Ohm-Farads (equals seconds) at higher capacitances. The Ohm-Farad expression allows a single figure to being used to describe the insulation performance of a given component family over a wide range of capacitance values. The leakage current is also dependent on the temperature. As the temperature increases, so does the leakage current.
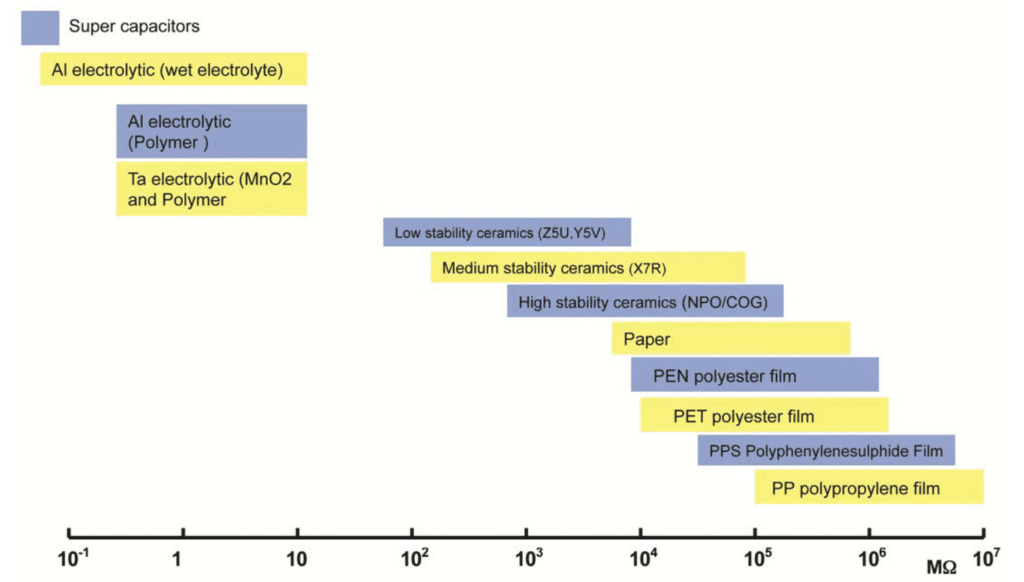
Figure 1. Values of capacitor types relative to dielectric Insulation Resistance (IR)
DCL leakage currents in electrolytic capacitors is also mentioned in the article here.
Dependence of leakage current on time
Charge/Discharge Behavior
When a DC voltage is applied to a capacitor connected in series with a resistor, the capacitor begins to charge at a rate according to the applied voltage, the state of charge relative to its final value, the series resistance, and its capacitance. The product of the resistance and capacitance is the circuit’s time constant (I = R x C). It is the time required to charge the capacitor by 63.2% of the difference between the initial and final values. Hence, the charge value plotted against time follows the curve shown in Figure 2. During this time, the charging current follows the red curve, also shown in Figure 2.
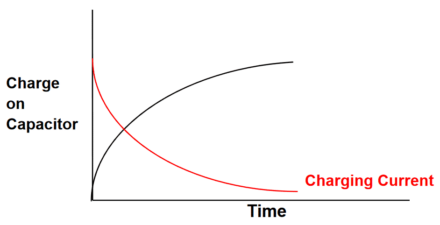
Figure 2. Capacitor charging and discharging curves
The charge on the capacitor at any time, t, is calculated by the following equation:
Q = C x V x [1 – e-t/RC]
The charging current decays according to the equation:
I = V/R x e-t/RC
Where e = 2.7182818, the so-called “natural number,” or the base of the natural logarithm, ln(x).
The leakage currents of some capacitors are dependent on time. The current is at its peak when the voltage is applied to a capacitor. The occurrence of this peak current depends on the construction of the capacitor. In the case of aluminum electrolytic capacitor, it is the forming characteristics of a capacitor and the internal resistance of the voltage source. When a capacitor is charged, its leakage current drops with time to a nearly constant value called operational leakage current. This small leakage current is dependent on both temperature and applied voltage.
Some capacitor technologies such as aluminium, tantalum, and film capacitors have self-healing properties. The self-healing process may have a significant effect on the leakage currents of the capacitors, while the exact mechanisms may be specific to the capacitor technology type. The time dependence of leakage currents is also caused by dielectric material type and structure. Other parameters that determine the value of this small current include the type of electrolyte, capacitance, and forming voltage of the anode. The leakage current of a ceramic capacitor does not change with time.
Dependence of leakage current on temperature
The leakage current of a capacitor is dependent on temperature. The level of dependency varies from one type of capacitor to another. For aluminium electrolytic capacitors, an increase in temperature speeds up the rate of a chemical reaction. This results in an increase in leakage current.
Compared to ceramic capacitors, tantalum capacitors have high leakage currents. The DC leakage current of a tantalum capacitor increases with an increase in temperature. The leakage currents of tantalum capacitors increase slightly when they are stored in a high-temperature environment. This small increase in leakage current is temporary and is reversed by applying rated voltage for a few minutes. In addition, the leakage current of a tantalum capacitor increases slightly when the component is exposed to high humidity. Voltage conditioning helps to reverse this temporary increase in leakage current.
Ceramic and film capacitors have small leakage currents relative to electrolytic capacitors. For multilayer ceramic capacitors (MLCCs), the intrinsic leakage currents increase exponentially with an increase in temperature. The properties of the dielectric material determine the insulation resistance of a film capacitor. For this type of capacitor, an increase in temperature causes a decrease in insulation resistance and an increase in leakage current.
Dependence of leakage current on voltage
A capacitor’s DC leakage current greatly depends on the applied voltage. For aluminium electrolytic capacitors, this current increases with an increase in operating voltage. As the operating voltage exceeds the rated voltage and approaches the forming voltage, the leakage current increases exponentially. When the voltage applied to an aluminium electrolytic capacitor exceeds the surge voltage, the tendency towards temperature rise, electrolyte degradation, formation of excess gas, and other secondary reactions increases. Due to this reason, operating an aluminium electrolytic capacitor beyond the rated voltage is not tolerable. The DC leakage current of an aluminium electrolytic capacitor drops sharply when the applied voltage is decreased below the rated voltage.
The leakage current of an aluminium electrolytic capacitor increases when the component is stored for a long period of time. Such capacitors are restored to their original characteristics through reconditioning. The process involves applying rated voltage to the capacitor for about half an hour.
For ceramic capacitors, the intrinsic leakage currents are greatly dependent on voltage—an increase in voltage results in a superlinear increase in intrinsic leakage current. The insulation resistance of a ceramic capacitor is independent of voltage.
DCL Myths
There are some common myths related to the DCL leakage current of capacitors that can still be heard today:
Myth 1: IR/DCL leakage current is due to the cracks in the dielectric.
This was one of the first imaginative theories of why dielectrics have a leakage current without a details understanding of the physical mechanisms inside the insulators. It is true that cracks and “imperfections” in dielectric structures can be a cause of leakage current increase and catastrophic failures on individual “faulty” components. On the other hand, it may not be the prime issue for the basic leakage current level – we have to understand the physical conductivity mechanisms that take place at the specific capacitor technology dielectric.
The details conductivity mechanisms description is beyond this lecture focus, but let’s simplify it that in a capacitor, the conductivity through the dielectric can be composed of three major mechanisms (all three are typical for electrolytic capacitors):
- Ohmic conductivity
- Poole Frankel mechanism – It can be imagined as electrons or holes “hopping” through a trap in the dielectric inner volume
- Tunneling mechanism – this dangerous zone should happen above the operational voltage. Under high electric field intensity, electrons/holes are accelerated to cross all the barriers with the risk of avalanche effect and catastrophic breakdown of the part resulting in a short circuit. So we can assume this is the main electrical breakdown mechanism
Another big influence on the value of DCL is the construction of the capacitor itself – namely, the electrical potential between the electrode systems and the insulating dielectric. Metal electrodes may have some sub-oxide layers that are semiconducting, and also electrolytes in electrolytic capacitors may exhibit rather semiconducting behavior – so, in-fact in many cases on capacitors, we are not faced with a simple Metal-Insulator-Metal structure, but more complex Metal-Insulator-Semiconductor systems, where interface barriers may play the leading role to the overall DCL leakage current values.
Myth 2: IR/DCL leakage current is a measure of the component reliability
This common myth is related to Myth 1 as the imagination was that the part with a higher leakage current also has a larger number of cracks, thus presenting a higher reliability risk.
As we learned in the Myth 1 mitigation above, the actual leakage current of a “standard” capacitor is due to its dielectric conductivity mechanisms and construction (electrical potential matching). DCL of statistically normally produced capacitors is not a measure of reliability. It was often confirmed that screening of DCL tail distribution is not improving the basic reliability numbers.
HOWEVER, the Change of DCL as the structural robustness to external load can be a measure of reliability. There is a number of proven screening methods that are part of specifications (MIL, ESA) or applied internally by manufacturers as their know-how where a certain (thermo)mechanical and electrical stress is applied with subsequent DCL screening to improve reliability level and sort-out suspicious parts.
Resource: Passive components Blog
- Flyback Converter Power Conversion Explained - June 26, 2024
- Addressing EMI of Common Mode Chokes - June 26, 2024
- How to Fix Power Supply EMC Issues - June 26, 2024
0 comments on Leakage Current Characteristics of Capacitors – Case Study